TDP-43 Model Overview
Cytoplasmic TDP-43 (or TDP43) aggregates are a hallmark of familial and sporadic ALS. While several transgenic (tg) mouse models of amyotrophic lateral sclerosis (ALS; also called motor neuron disease [MND]) with TDP-43 aggregation exist, they each have their respective strengths and weaknesses.
At Biospective, we use both the original and modified versions of the rNLS8 (or ΔNLS; delta NLS) ALS mouse model of TDP-43 proteinopathy:
- Original mouse model ("Off Dox"): rapidly progressing (weeks)
- Biospective mouse model ("Low Dox"): slower progressing (months)
Significant advantages of these TDP-43 mice for ALS researchers include:
- Mislocalization of TDP-43 to the cytoplasm
- Progressive motor deficits
- Motor neuron degeneration & regional brain atrophy
- Neuroinflammation
- Brain, spinal cord, and neuromuscular junction pathology
The model time course is predictable and the measures of disease progression are highly reproducible.
TDP-43 Mice Generation
rNLS8 (NEFH-hTDP-43-ΔNLS) double transgenic ALS mice are generated by breeding mice having the NEFH-tTA transgene with mice having the tetO-hTDP-43-ΔNLS transgene. This TARDBP model was originally developed and reported by Walker et al. (Acta. Neuropathol., 130: 643-670, 2015). It is a model of amyotrophic lateral sclerosis (ALS) or motor neuron disease (MND). It can also be used as a TDP-43 pathology model of frontotemporal dementia (FTD) or frontotemporal lobar degeneration (FTLD).
These TDP-43 transgenic mice are maintained on a Dox diet during breeding and the initial aging period (typically 5 to 12 weeks-of-age). The mice are then changed from a Dox diet to a standard diet ("Off Dox" model) or an alternate protocol developed by Biospective ("Low Dox" model) to allow for human TDP-43 expression. An interesting feature of this model is that pathologic and functional recovery can be achieved by putting mice back on a Dox diet.
Our Validated TDP-43 Transgenic Mice Measures
- Body weight
- Motor scoring (clasping, grill agility, paralysis, tremor)
- Grip strength test
- MRI brain atrophy to measure neurodegeneration (Read More in our Presentation - Brain atrophy analysis in rodent models of neurodegenerative diseases)
- In vivo muscle electrophysiology (CMAP)
- Muscle atrophy measured by computerized tomography (CT)
- Neurofilament Light measures in plasma & CSF
- Immunohistochemistry & multiplex immunofluorescence
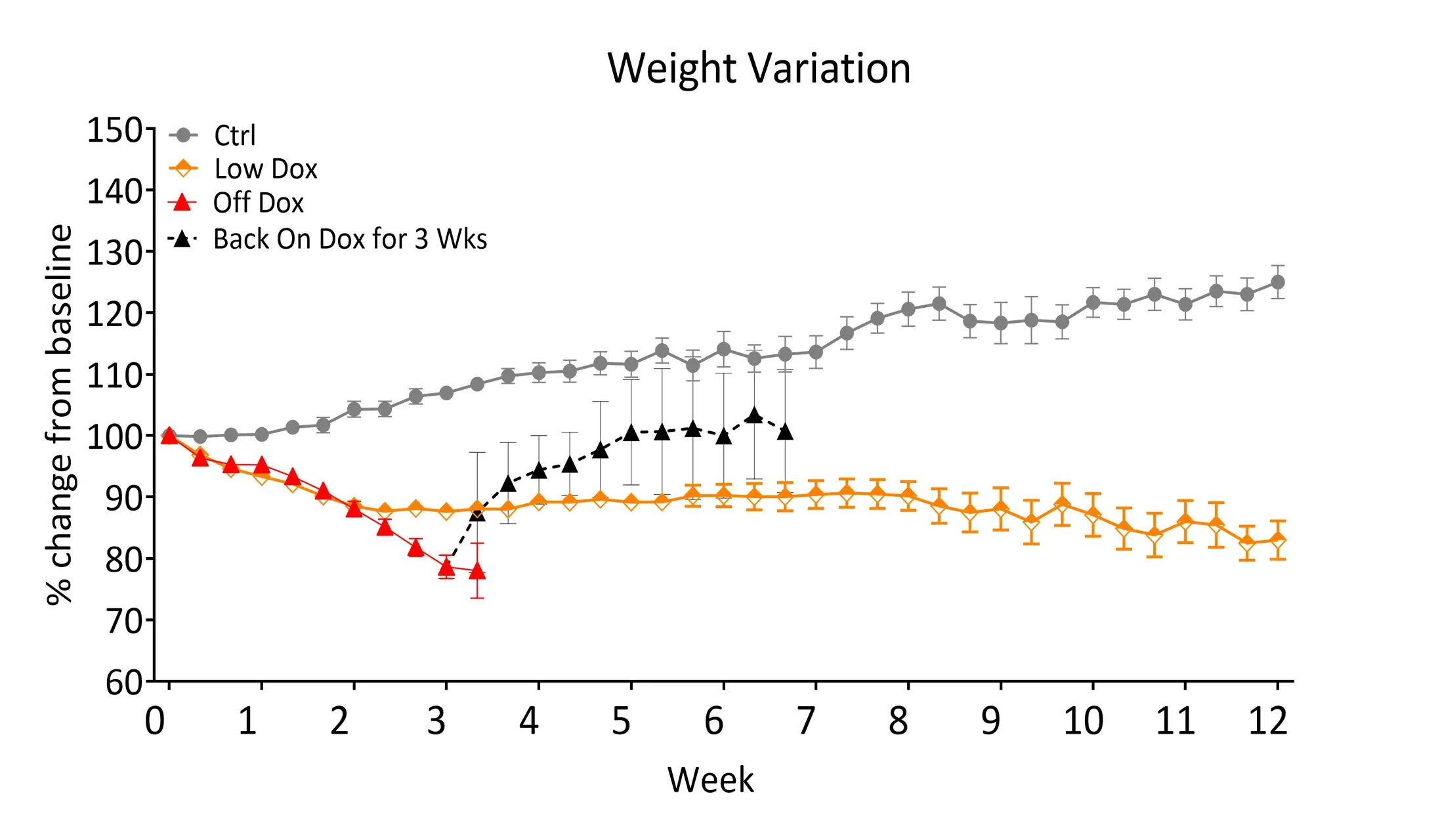
Learn more about our characterization of this model, our validated measures, and our Preclinical Neuroscience CRO services.