What is the neuromuscular junction?
The neuromuscular junction (NMJ) is a specialized synapse that allows communication between motor neurons and skeletal muscle fibers, enabling muscle contractions necessary for movement. The NMJ is comprised of four major components: presynaptic motor neurons, postsynaptic muscles, terminal Schwann cells, and kranocytes (Verma, 2022). The presynaptic component includes nerve terminals, where motor neuron axons branch and release acetylcholine (ACh) neurotransmitters in response to electrical signals. These neurotransmitters bind to ACh receptors (AChR) in the junctional folds of the muscle membrane, also known as the motor endplate, triggering an action potential that drives muscle contraction. Terminal Schwann cells are non-myelinating cells that cap the presynaptic nerve terminals and extend processes over the synaptic cleft. They are critical for NMJ formation, maturation, maintenance, and regeneration (Sanes, 1999; Alhindi, 2021). Kranocytes are fibroblast-like cells capping the terminal Schwann cells and the entire endplate area, and they also contribute to synaptic regeneration (Sugiura, 2011). The precise spatiotemporal organization of these NMJ components is essential for efficient neuromuscular transmission, with disruptions potentially leading to functional impairment, denervation, muscle weakness, and/or paralysis.
Schematic representation of the main components of the neuromuscular junction. Abbreviations: Neuromuscular Junction (NMJ), Acetylcholine (ACh), ACh Receptors (AChR).
The NMJ is an experimentally interesting synapse model because it is accessible in a wide variety of animal models, including, but not limited to, mice, rats, and drosophila. Unlike the motor neuron soma located in the spinal cord, the NMJ is located in peripheral tissues, making it more accessible for imaging, biopsies, and experimental manipulation. Additionally, numerous publicly available tools enable visualization and quantification of NMJs, making them an ideal system for studying the mechanisms underlying neurodegenerative disorders (Murray, 2010).
How are NMJs affected in ALS & animal models of ALS?
Various studies have shown that NMJ degeneration occurs before the onset of clinical symptoms and motor neuron loss in rodent ALS models (Kennel, 1996; Frey, 2000; Verma, 2022). These observations have led researchers to propose that ALS is likely a “dying back” motor neuropathy, where neuronal degeneration starts at the distal ends of the axons before affecting the motor neuron cell bodies (Frey, 2000). Given the NMJ's role as an early and critical site of pathology in ALS, it represents a promising therapeutic target for preserving neuronal integrity and potentially delaying or preventing disease progression.
Morphological changes in NMJs have been consistently observed in animal models of ALS. Increased axonal fragmentation and loss of axonal innervation are commonly reported in NMJs of ALS mouse models (Pigna, 2019; Guerra San Juan, 2022; Alhindi, 2023). Although axonal sprouting and reinnervation often occur as compensatory mechanisms, these responses are typically incomplete or transient (Schaefer, 2005; Carrasco, 2016; Martineau, 2018). Further, altered endplate morphology, such as shrinking of postsynaptic endplates and disrupted arrangement of acetylcholine receptors, is frequently observed in ALS animal models (Sakowski, 2012; Picchiarelli, 2019; Mejia Maza, 2021; Alhindi, 2022; Verma, 2022).
In humans, studies identifying early changes at the NMJ are limited due to the difficulty of obtaining presymptomatic samples. This issue has created challenges in validating findings from animal models. Post-mortem analysis of human tissues, however, has revealed significant denervation and reduced nerve terminal presence in ALS patients (Tsujihata, 1984; Maselli, 1993). More recently, researchers reported abnormal electromyography (EMG) results, fragmented synaptic gutters, sparsely branched terminal axonal arborization, and altered terminal Schwann cell morphology in ALS patients (Bruneteau, 2015).
What tools are available to quantify morphological changes in NMJs?
Multiplex immunofluorescence (mIF) is the preferred tool for analyzing the morphology of neuromuscular junctions due to its ability to provide high-resolution, specific staining of the various NMJ subcomponents. It is compatible with a wide range of experimental models, including tissue sections, whole-mount preparations, and even cultured cells. Typically, the presynaptic neuronal architecture of the NMJ is labeled using antibodies against neurofilament and synaptic vesicles, while postsynaptic acetylcholine receptors are labelled with α-bungarotoxin.
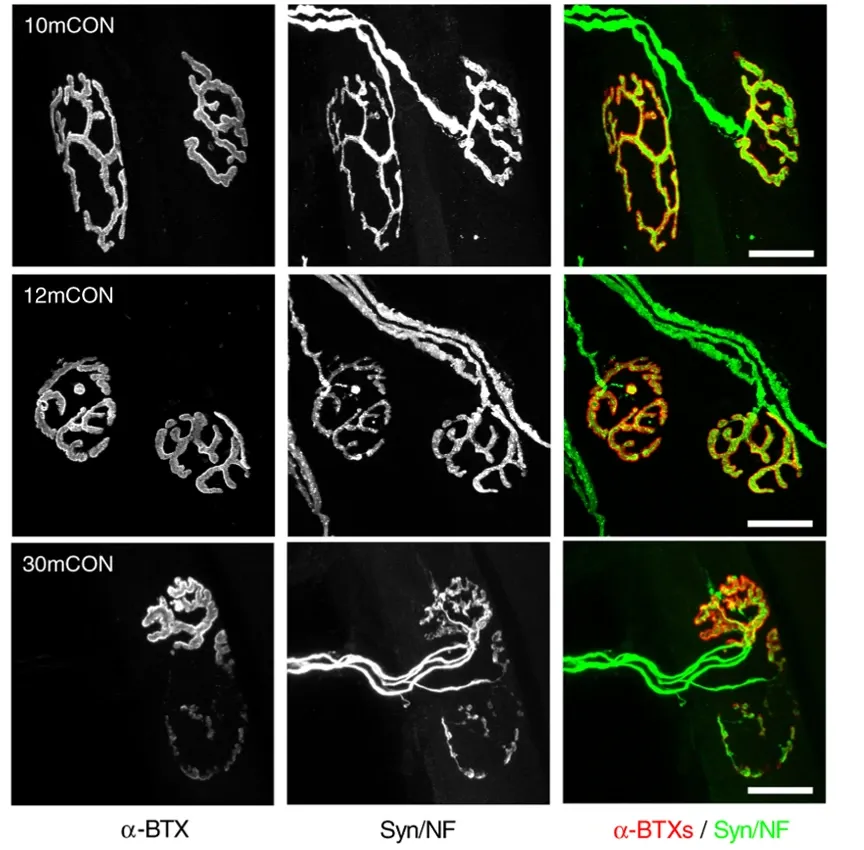
Representative whole-mount images of the presynaptic (neurofilament/synaptophysin) and postsynaptic (acetylcholine receptors [AChRs] stained using alpha-bungarotoxin) in extensor digitorum longus (EDL) muscle for 10-month-old control (10mCON), 12-month-old control (12mCON), and 30-month-old (30mCON) mice. Figure and caption adapted from Ham et al. (Ham, 2020) under the Creative Commons Attribution License.
NMJs can be visualized using confocal z-stack projections or widefield fluorescence microscopy, with the former typically offering better spatial resolution. Due to the complex, plaque-like geometries of NMJs, great care must be given to analyze only en-face NMJs and to reject oblique NMJs that can appear compressed or distorted due to the viewing angle, making it difficult to assess their true size, shape, and structural integrity.
Various publicly-available image processing tools exist for quantifying NMJ morphology. A widely used ImageJ plugin, “NMJ-morph”, applies simple thresholding algorithms to segment presynaptic and postsynaptic components from fluorescence channels. It generates a range of morphological metrics for the presynaptic axons and the motor endplate, such as area, diameter, compactness, and fragmentation. NMJ-morph has been employed in studies of diverse pathology models, including Charcot-Marie-Tooth disease (Cipriani, 2018), CHCHD10-encoded mitochondrial myopathy (Genin, 2019), myasthenic syndrome (McMacken, 2019), ALS mouse models (Pigna, 2019; Alhindi, 2023; Vieira de Sá, 2024), cancer cachexia (Boehm, 2020), and sarcopenia (Ham, 2020). More recently, the same group that developed NMJ-morph have released an updated algorithm called “automated NMJ-morph” (aNMJ-morph), which streamlines and expedites data acquisition to improve the relatively low throughput of NMJ-morph and its associated steep learning curve (Minty, 2020). This enhanced algorithm has been applied in studies on YAP1/TAZ-TEAD signaling (Gessler, 2024), human vocalis muscle (Tracicaru, 2024), gut microbiota (Cescon, 2024), and peripheral nerve injuries (Mehrotra, 2024).
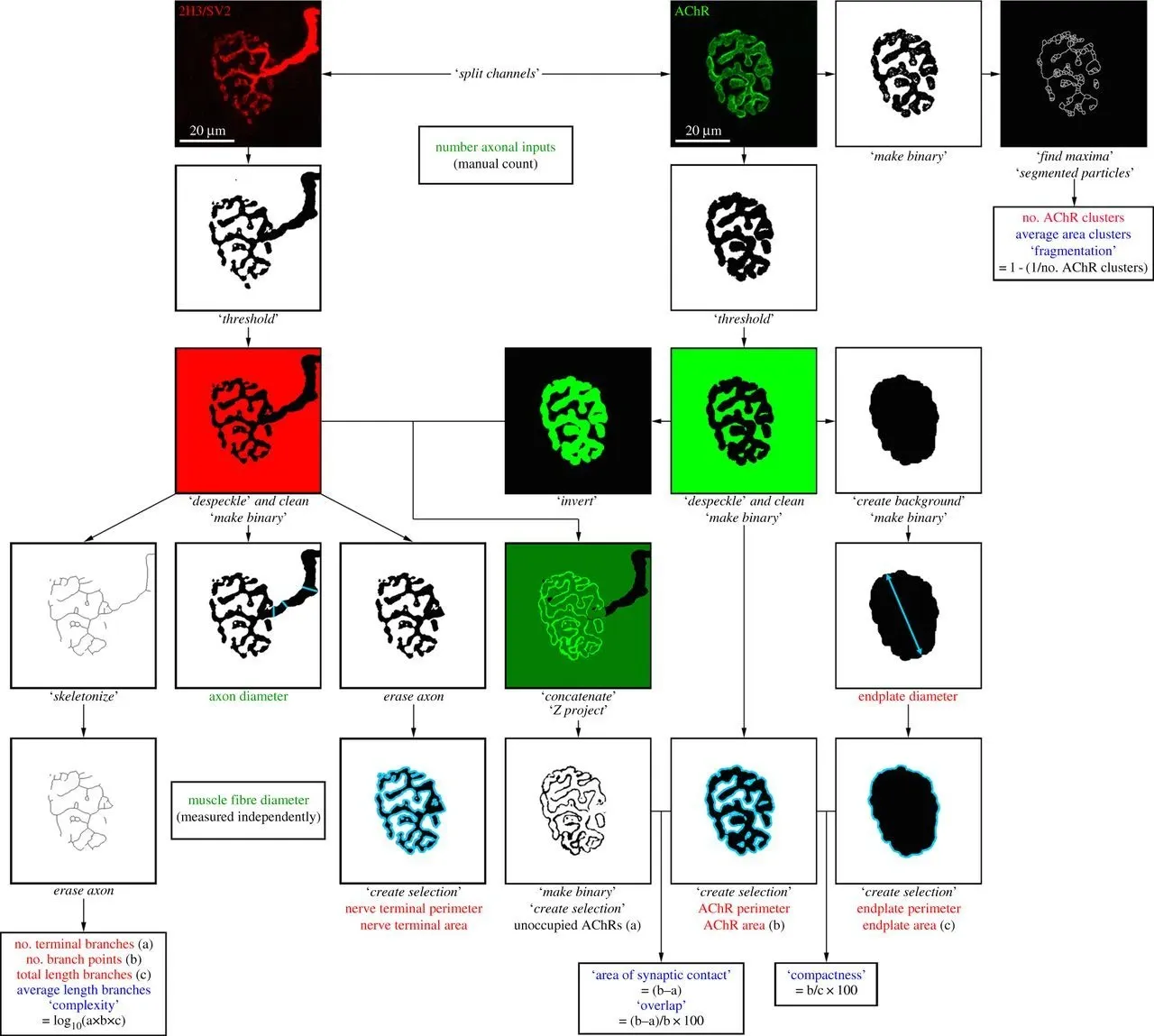
Overview of the NMJ-morph platform. Flowchart demonstrating the sequence of analyses for each NMJ. The workflow comprises 21 morphological variables: the 11 ‘core variables’ are shown in red, the 7 ‘derived variables’ in blue, and the 3 ‘associated nerve and muscle variables’ in green. Specific operations within NMJ-morph are shown in italics. Figure and caption reproduced from Jones et al. (Jones, 2016) under the Creative Commons Attribution License.
Since NMJ-morph and similar ImageJ plugins rely on manual thresholding, they are prone to inter-laboratory and inter-rater variability. To address this issue, Mejia Maza et al. (Mejia Maza, 2021) have developed “NMJ-analyser”, which introduces experiment-wide normalization methods to minimize batch effects and enables fully automatic threshold selection. Unlike its predecessors, NMJ-analyser operates on 3D stacked raw images (z-slice) instead of 2D maximum projections, allowing analysis of all NMJs rather than restricting to en-face views. It also incorporates a Random Forest machine learning algorithm to classify the innervation status of NMJs between “fully innervated”, “partially innervated”, and “denervated” based on the wide range of morphological features it computes. NMJ-analyser was shown to detect changes at the NMJ with higher sensitivity compared to NMJ-morph (Mejia Maza, 2021).
Our team would be happy to answer any questions about Neuromuscular Junction (NMJ) Morphology or provide specific information about the ALS models that we use for therapeutic efficacy studies.
Discover more about our ALS Models
Related Content
Up-to-date information on Neuromuscular Junction (NMJ) Morphology and best practices related to the evaluation of therapeutic agents in ALS animal models.
ALS Mouse Models & Spinal Motor Neurons
An overview of the involvement of spinal motor neurons in disease progression in mouse models of Amyotrophic Lateral Sclerosis (ALS).
ALS Mouse Models for Drug Development
A guide to the most effective use of research animal models (mouse & rat models) of Amyotrophic Lateral Sclerosis (ALS) for preclinical testing of therapeutics.
Microglia Morphology in ALS, Alzheimer's Disease & Parkinson's Disease
An overview of microglial morphological analysis and the applications to neurodegenerative disease research and drug discovery & development.
Brain Atrophy Analysis in Mouse Models of Neurodegeneration
Automated in vivo MRI-based quantitative brain atrophy measures (regional brain volumes and cortical thickness) from mouse models of ALS & Parkinson's disease.
TDP-43 ΔNLS (rNLS8) Mice for ALS Drug Development
This resource provides information about the use of the ΔNLS (deltaNLS, hTDP-43ΔNLS, hTDP-43DeltaNLS, dNLS, TDP43 NLS, rNLS8) TDP-43 transgenic mouse model of ALS for preclinical therapeutic studies.