What is autophagy?
The term autophagy, derived from the Greek word meaning to eat oneself, was coined by Belgian biochemist Christian de Duve in 1966 to describe a lysosomal-mediated degradative process within eukaryotic cells that removes and recycles cellular debris—from bacteria and viruses to abnormal proteins and injured organelles (Cai, 2022). These components are used for the synthesis of new molecules, allowing cells to repair and rebuild themselves in order to survive and function more efficiently (Nixon, 2013).
In eukaryotic cells, three main autophagic pathways have been identified: macro-autophagy, micro-autophagy, and chaperone-mediated autophagy. While these pathways converge on lysosomal degradation, they differ in the type of cellular cargo they target, and the underlying mechanisms they use for this process (Nakatogawa, 2009; Kesidou, 2013).
Due to the post-mitotic nature of neurons, autophagy appears to play a protective role in neuronal homeostasis and integrity (Bhukel, 2019; Stavoe, 2019). Both inherent and stress-induced pathways for autophagy are present in neurons, with the latter triggered by hypoxia, excess of damaging free radicals, and mitochondrial damage (Lee, 2011; Stavoe, 2019). Beyond these pathways, autophagy has other specialized roles in the central nervous system—it aids with the myelination process, which is essential for neuronal signaling, and regulates the inflammatory response of microglia. Dysregulation of these processes can lead to irreversible damage in the CNS and neuronal death (Su, 2016).
To investigate the role of autophagy in neurons, researchers employ techniques, like electron microscopy (EM), which allow visualization of autophagic compartments, such as membrane-bound vesicles that traffic cellular components to the lysosome. Immunoblot analysis of the microtubule-associated protein LC3, specifically LC3-II, is another widely-used approach for quantifying autophagy within cells, as its levels correlate with the formation of autophagosomes (Klionsky, 2016). In vivo studies with GFP-LC3 transgenic mice also enable systematic observation and analysis of autophagic processes (Mizushima, 2004).
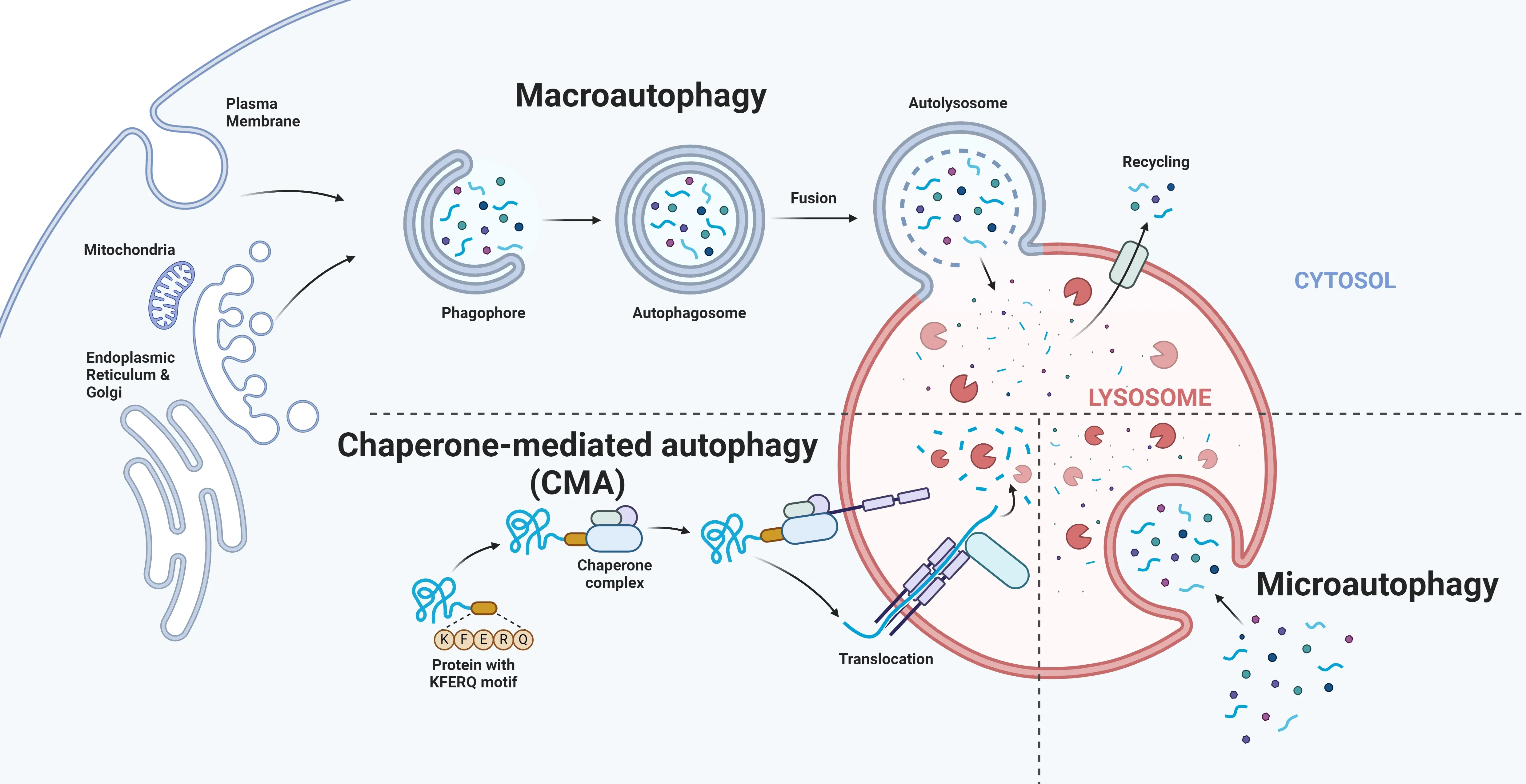
In eukaryotic cells, three main autophagic pathways—macroautophagy, microautophagy, and chaperone-mediated autophagy—are responsible for the removal and recycling of cellular debris. Despite the distinct mechanisms involved, these pathways converge at the lysosome.
Which neurodegenerative diseases are associated with impaired autophagy?
Impaired autophagy is closely associated with the pathology of several neurodegenerative diseases, which are characterized by the aggregation of abnormal proteins within neurons (Kesidou, 2013). Mutations in core autophagy-related genes encoding proteins critical for autophagic pathways have been implicated in Alzheimer’s (AD), Parkinson’s disease (PD), amyotrophic lateral sclerosis (ALS), and Huntington’s disease (HD) (Nah, 2015; Deng, 2017; Menzies, 2017).
Mouse models have offered deeper insights into this connection. For example, when Hara et al. (Hara, 2006) studied the role of autophagy in neural cells in the absence of disease-associated mutant proteins using neural cell-specific Atg5 knockout mice, histological analysis of brains from these mice revealed neuronal loss, degeneration in cerebellar Purkinje cells, and the accumulation of ubiquitin-positive inclusion bodies.
Beclin-1, a well-established regulator of autophagy, has been implicated in several neurodegenerative diseases (Pickford, 2008; Son 2012). In AD, Beclin-1 levels are reduced in the brain early in the disease process, disrupting autophagic flux (Pickford, 2008). In HD, the mutant Huntingtin gene binds to Beclin-1, impairing its function (Shibata 2006; Wu, 2012). Beclin-1 also facilitates the degeneration of alpha-synuclein, which forms toxic aggregates within neurons in PD. When Spencer et al. (Spencer, 2009) delivered lentivirus-expressed Beclin-1 into the brains of alpha-synuclein transgenic mice, the group observed improved synaptic and dendritic pathology, and reduced alpha-synuclein accumulation in the limbic system.
Mutations in ALS-linked genes—SOD1 and TDP-43—have been linked to failures across different stages of the autophagy process (Lee, 2015). Rudnick et al. (Rudnick, 2017) explored the role of autophagy in motor neurons at different stages of the ALS disease process by crossing Atg7 knockout mice with an ALS mouse model. Inhibition of motor neuron autophagy in SOD1-G93A mice accelerated the denervation of fast muscle fibers and the onset of tremor, suggesting that autophagy plays a protective role in maintaining muscle-nerve connections during the early stages of ALS.

A representation of the molecular hallmarks of neurodegeneration: protein misfolding, protein aggregates, autophagy disruptions, oxidative stress, neuron dysfunction, and apoptotic cell death. Figure and caption are reproduced from De Chiara et al. (De Chiara, 2012) under the Creative Commons Attribution License.
What is the relationship between autophagy and misfolded proteins?
Autophagy plays a complementary role to the proteasome in degrading misfolded proteins. Disruptions in autophagic activity are closely linked to the accumulation of toxic protein aggregates within neurons, leading to neurodegeneration (Nixon, 2006; Williams, 2006; Zhang, 2007; Son 2012). Studies in mouse models of neurodegenerative diseases have shown that enhancing autophagic activity through rapamycin promotes the clearance of these pathological protein aggregates (Caccamo, 2010). However, it is important to note that the relationship between misfolded proteins and autophagy is bi-directional—misfolded proteins can interfere with autophagic pathways, creating a feedback loop that exacerbates disease progression (Cuervo, 2004; Orenstein, 2013).
Amyloid-beta
Autophagy plays a key role in the production, secretion, and clearance of intracellular amyloid-beta. Amyloid-beta peptides are generated in autophagosomes during degradation of amyloid precursor protein (APP)-rich organelles, like Golgi-derived vesicles and endosomes (Nixon, 2007). Studies in mouse models suggest that the secretion of these peptides from neurons is facilitated by autophagy. When Nillson et al. (Nillson, 2013) crossed APP transgenic mice with mice lacking critical autophagy mechanisms in their forebrain, the mice had fewer amyloid-beta plaques compared to those with functional autophagy. This reduction, the team reported, was due to the inability of neurons to secrete amyloid-beta in the absence of autophagy.
Tau
This microtubule-associated protein, implicated in AD, is a key regulator of autophagy. Increased Tau phosphorylation destabilizes microtubules, impairing their ability to transport cellular cargo for degradation via autophagy (Esteves, 2019). In addition, accumulation of tau blocks autophagosome-lysosome fusion, inhibiting autophagic flux and perpetuating its own accumulation—a vicious cycle in AD (Feng, 2019).
Alpha-synuclein
Alpha-synuclein is degraded not only by the proteasome, but also through chaperone-mediated autophagy and macro-autophagy (Vogiatzi, 2008; Vidal, 2014; Frake, 2015). Mutations in autophagy-regulating proteins, such as LRRK2, PINK1, and PARKIN, promote the misfolding and accumulation of alpha-synuclein (Beilina, 2015; Hou, 2021; Zhang, 2022).
TDP-43
TAR DNA binding protein 43 (TDP-43) is an RNA/DNA binding protein. Stimulating autophagy in neurons can increase TDP-43 turnover and reduce its mislocalization from the nucleus to the cytoplasm in neurons and microglia—a hallmark of ALS and FTD pathology (Barmada, 2014).
Our team would be happy to answer any questions about autophagy in neurodegenerative diseases or provide specific information about the AD, ALS, and PD models we use for therapeutic efficacy studies.
Discover more about our Neurodegenerative Diseases Models
Related Content
Up-to-date information on autophagy in neurodegenerative diseases and best practices related to the evaluation of therapeutic agents in animal models of neurodegenerative diseases.
Amyloid-β Plaque Analysis in Alzheimer's Disease
Overview of methods to classify & quantify Aβ plaques in brain tissue sections from humans & Alzheimer’s disease animal models (transgenic mice & rats).
ALS Mouse Models & Spinal Motor Neurons
An overview of the involvement of spinal motor neurons in disease progression in mouse models of Amyotrophic Lateral Sclerosis (ALS).
Microglia Morphology in ALS, Alzheimer's Disease & Parkinson's Disease
An overview of microglial morphological analysis and the applications to neurodegenerative disease research and drug discovery & development.