What is the TDP-43 ΔNLS transgenic mouse model?
The TDP-43 ΔNLS model, also known as deltaNLS, delta NLS, hTDP-43ΔNLS, hTDP-43DeltaNLS, NEFH-hTDP-43ΔNLS, dNLS, TDP43 NLS, rNLS, and rNLS8, is a transgenic mouse originally reported by Walker and colleagues in 2015. The key, unique characteristic of this model is that the expressed human TDP-43 protein has a defective nuclear localization signal (NLS) that prevents translocation to the nucleus (where it would normally localize and function). The resulting accumulation in the neuronal cytoplasm leads to the formation of aggregates, including phosphorylated TDP-43 aggregates, similar to those observed in ALS patients.
In this model, the expression of hTDP-43ΔNLS is controlled by the neurofilament heavy (NEFH) promoter, thereby limiting it to neurons. In a predecessor model, reported by Igaz and colleagues, the expression was under the Camk2a promoter, which led to accumulated cytoplasmic TDP-43 in the brain, but with minimal pathological TDP-43 aggregates and lack of an ALS-like phenotype. The expression is "regulatable" (hence "rNLS") by the tetracycline analog, doxycycline (Dox). The rNLS8 double transgenic line, with expression of hTDP-43ΔNLS in both the brain and spinal cord, is generated by breeding the individual transgenic lines: NEFH-tTA (Line 8; NEFH promoter directing tetracycline-controlled transactivator protein [tTA] expression) and tetO-hTDP-43ΔNLS (Line 4; tetracycline operator promoter [tetO] directing the expression of hTDP-43ΔNLS). The double transgenic mice are maintained on a Dox diet during breeding and the first several weeks-of-life (typically 5-12 weeks-of-age) to suppress the expression of hTDP-43ΔNLS. Disease induction is initiated by switching to standard chow (the "Off Dox" model). Note that mice maintained on the Dox diet ("On Dox" mice) can serve as a control group as they do not show signs of disease.
A key feature of this model is the ability to obtain a pathologic and functional recovery by reinstating the Dox diet. The halting and reversibility of the disease progression in this model demonstrates that disease modification is possible via mediation of TDP-43 expression and pathological aggregates, thereby making it an attractive model for ALS drug development.
The original ΔNLS model is rapidly progressing with weight loss and motor symptoms typically appearing within approximately one week from the removal of Dox to death within several weeks. As an alternative that is better suited for most therapeutic efficacy studies, our team developed a slower progressing version using an alternative Dox protocol. In our "Low Dox" model, a similar phenotype is produced, but evolves over a longer period of time with mice surviving to at least 3 months after model induction.
What are key features of human ALS that are present in TDP-43 ΔNLS mice?
ALS is a fatal neurodegenerative disease that affects numerous systems in humans, including the central nervous system (CNS), peripheral nervous system (PNS), autonomic nervous system (ANS), muscle, and immune system. As summarized in the infographic below, the ΔNLS model has been demonstrated to recapitulate a number of these features, with others (e.g. ANS and immune system) still to be explored.
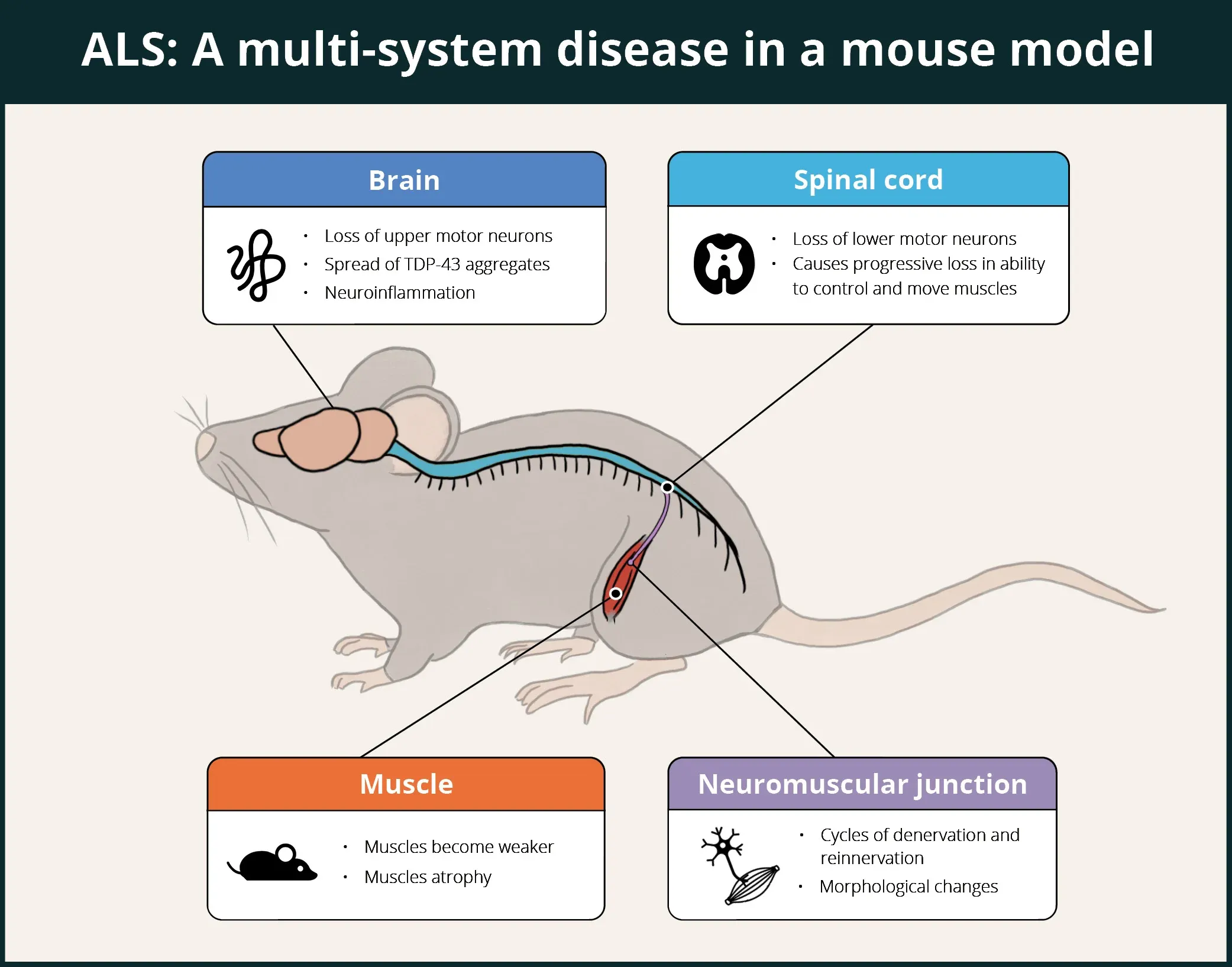
TDP-43 ΔNLS mice demonstrate multi-system involvement, including brain, spinal cord, neuromuscular junctions (NMJ), and muscles, thereby making it a good model for evaluation of putative therapeutic agents for ALS.
Click to copy link
In the brain, ΔNLS mice show cytoplasmic TDP-43 aggregates (including phosphorylated TDP-43), neurodegeneration, impaired glymphatic function, neuroinflammation (microgliosis and astrogliosis), and a proteomic signature that correlates with that of post-mortem human brain tissue from patients with TDP-43 proteinopathies.
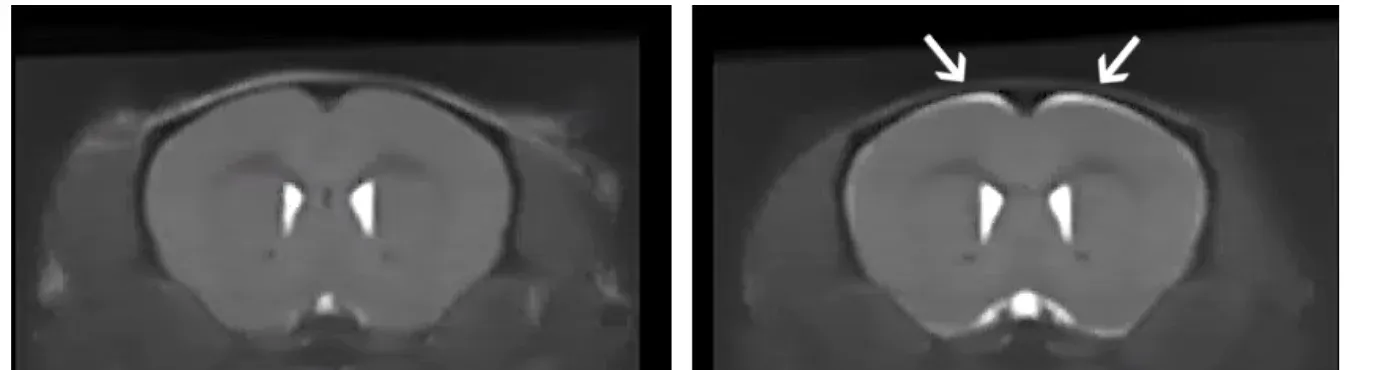
Anatomical MRI from control (left) and ΔNLS mice off Dox for 3 weeks (right). Note the bright signal in specific regions showing brain atrophy in the ΔNLS mice.
In terms of neuroinflammation, microglia play an intriguing role in this model. Spiller et al. found that after TDP-43 expression was halted by putting mice back on Dox, microglia transiently proliferated and changed their morphology and gene expression, facilitating the clearance of the cytoplasmic TDP-43. When microglia were depleted, the recovery was attenuated. Hunter et al. performed a transcriptomic analysis during the progression and recovery phases, and found that differentially expressed genes were associated with chemotaxis, phagocytosis, inflammation, and production of neuroprotective factors. Swanson et al. found that microglia are phagocytic at early stages of the disease and transition to a dysfunctional state at later stages, and that these functional states are driven by phosphorylated TDP-43 aggregation.
In the spinal cord, Spiller et al. found that slow motor neurons are resilient while fast fatigable motor neurons are lost, and axonal dieback occurs first from fast-twitch muscle fibers, while slow-twitch fibers remain innervated. These findings in the mouse model mirror the selective patterns of motor neuron degeneration in human disease.
Walker et al. reported significant early and progressive neuromuscular junction (NMJ) denervation. Hur et al. demonstrated that motor neuron identity is responsible for the susceptibility of NMJs to TDP-43 pathology, and that slow motor neurons can drive the recovery of motor systems as a function of their resilience to TDP-43 associated neurodegeneration. Several papers, including Spiller et al., have reported reduction of compound muscle action potential (CMAP) amplitude.
In terms of muscle involvement, muscle weakness, grouped fiber atrophy and centralized nuclei have been found in muscle fibers, and loss of muscle mass in the gastrocnemius and tibialis anterior muscles have been reported by Walker and colleagues. Tsitkanou et al. found hTDP-43 cytoplasmic accumulation of TDP-43 and oligo A11 β-amyloid in denervated muscle fibers in the tibialis anterior, quadriceps, and diaphragm muscles. They also found atrophy of the tibialis anterior, quadriceps, and gastrocnemius muscles, an upregulation of markers of myogenic and neuromuscular junction (NMJ) stress, impaired motor function and muscle force production, and dysregulation of several proteins related to mitochondrial complexes.
Our group has used non-invasive computed tomography (CT) image to demonstrate selective muscle atrophy, which most significantly affects the hindlimb gastrocnemius muscle, in our "Low Dox" mouse model.
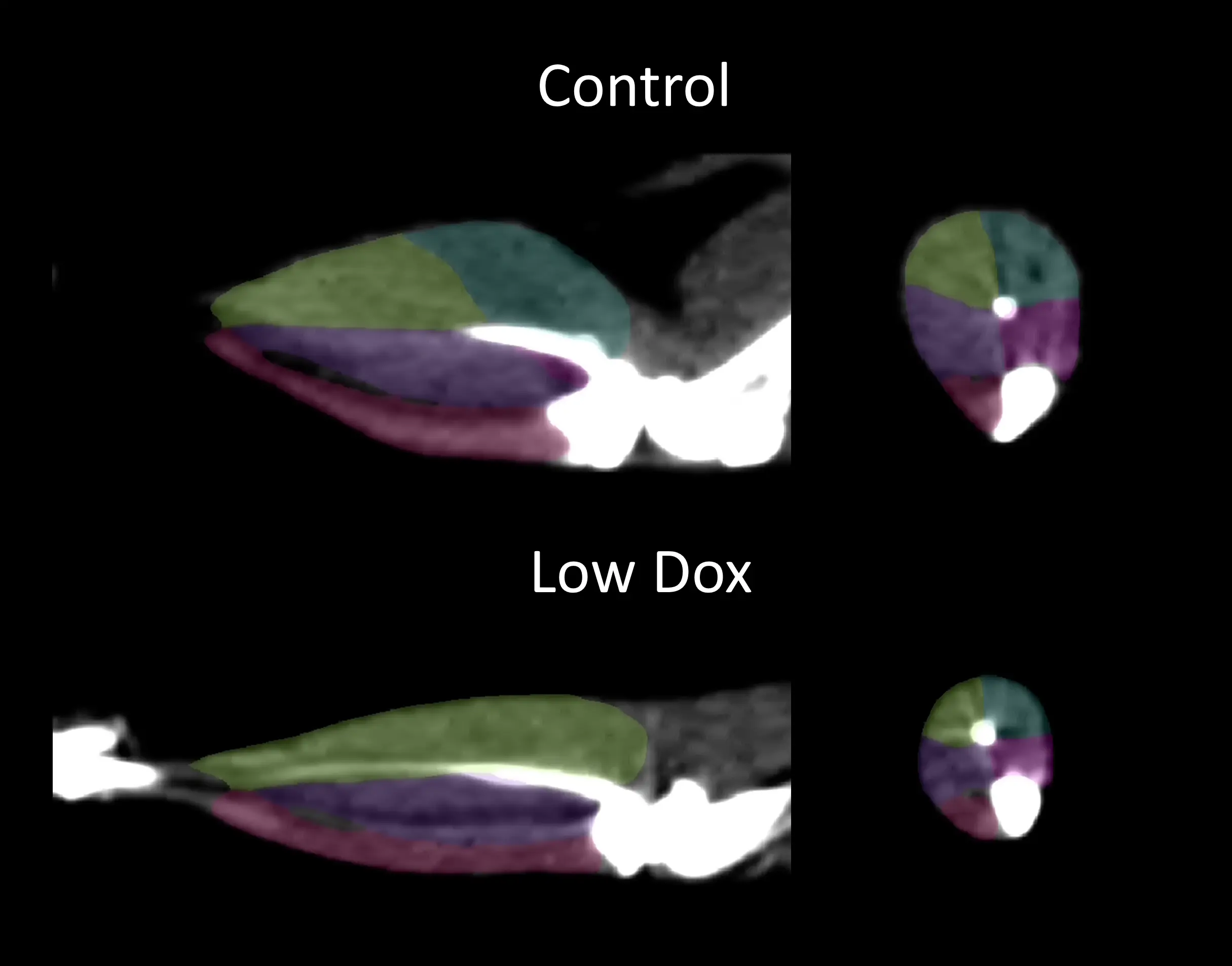
CT image with segmentation of the hindlimb muscles from Control (On Dox) and "Low Dox" TDP-43 ΔNLS mice; medial gastrocnemius = blue; lateral gastrocnemius = yellow; posterior tibialis = purple; anterior tibialis= pink; soleus = magenta.
3D volume rendering of the medial (blue) and lateral (yellow) gastrocnemius muscles from Control and "Low Dox" TDP-43 ΔNLS mice.
What measures have been used to assess therapeutic efficacy in the TDP-43 ΔNLS ALS mouse model?
Several groups have shown disease modification via therapeutic intervention in this mouse model. Young et al. have demonstrated the ability of a small molecule PIKfyve inhibitor, AIT-101 (INN: apilimod, aka LAM-002A), to decrease the loss of body weight, reduce motor deficits (including hindlimb clasping, hindlimb paralysis, and grill agility), along with accompanying decreases in plasma and CSF neurofilament light (NfL) levels, TDP-43 aggregates (by IHC), and neuroinflammation (by GFAP IHC) in Biospective's Low Dox version of the rNLS8 model.
Stomakhina et al. found reduction in plasma and CSF neurofilament light (NfL) levels, as well as reduction in acid sphingomyelinase (ASM) activity in the caudal cortex in rNLS8 mice treated with VRG50304 over a 28 day period. Droppelmann et al. found that intracerebroventricular injections of AAV9/NF242 N-terminal fragment of rho guanine nucleotide exchange factor [RGNEF]) in the rNLS8 model improved lifespan and motor phenotype (hindlimb clasping, open field, gait [CatWalk]), and decreased neuroinflammation markers (GFAP and Iba-1).
Our team would be happy to answer any questions about the TDP-43 ΔNLS ALS mouse model or provide specific information about the models that we use for therapeutic efficacy studies.
Discover more about our ALS Models
Related Content
Up-to-date information on Amyotrophic Lateral Sclerosis and best practices related to the evaluation of therapeutic agents in ALS animal models.
ALS Mouse Models for Drug Development
A guide to the most effective use of research animal models of Amyotrophic Lateral Sclerosis (ALS) for preclinical testing of therapeutics.
Brain Atrophy Analysis in Rodent Models of Neurodegeneration
Brain atrophy is a hallmark of many neurodegenerative diseases. MRI is routinely used in clinical trials to non-invasively measure volumetric and cortical thickness changes. We have applied these translational imaging biomarkers to mouse models of ALS and Parkinson’s disease.