What is the role of TNF-α in the central nervous system (CNS)?
Tumor necrosis factor-alpha (TNF-α) is a pro-inflammatory cytokine that plays a crucial role in regulating inflammation and immune responses. In the central nervous system (CNS), TNF-α has a dual function, contributing positively under healthy conditions, but potentially causing harm when dysregulated in diseased states. Under homeostatic conditions, TNF-α supports neuroplasticity, myelination, and tissue repair. However, when its levels become elevated, it can lead to detrimental effects, such as excitotoxity, chronic inflammation, and breakdown of the blood-brain barrier (BBB). TNF-α exists in both soluble and transmembrane forms, and acts through two main receptors: TNF receptor type-1 (TNFR1) and TNF receptor type-2 (TNFR2). Its expression is tightly regulated by various transcription factors, notably nuclear factor kappa-light-chain-enhancer of activated B cells (NF-κB). When activated, NF-κB not only promotes TNF-α production, but also triggers the expression of other pro-inflammatory cytokines, such as IL-6 and IL-1β. This interaction between TNF-α and NF-κB creates a positive feedback loop, which amplifies the inflammatory response and sustains the immune reaction (Gonzalez Caldito, 2023).
Microglia, the resident immune cells of the CNS, are the main source of TNF-α during neuroinflammation, although astrocytes can also contribute to its release (Olmos, 2014). In neurodegenerative diseases, microglial reactivity, along with other states like microglial senescence and the senescence-associated secretory phenotype (SASP), results in increased production of TNF-α and other pro-inflammatory cytokines, sustaining a chronic inflammatory environment. Inflammatory cytokines, such as interferon-gamma (IFN-ϒ), can further stimulate microglia to release TNF-α, thereby exacerbating inflammation. This ongoing inflammatory response accelerates the progression of neurodegenerative diseases, such as Alzheimer's disease (AD), Parkinson’s disease (PD), and amyotrophic lateral sclerosis (ALS).
Therapeutic strategies targeting TNF-α have shown potential in treating CNS autoimmune diseases, and may offer a way to modify the neuropathological features of neurodegenerative diseases (Torres-Acosta, 2020; Gonzalez Caldito, 2023). However, while anti-TNF-α therapies can reduce inflammation, their effects are complex and can sometimes lead to unintended consequences, such as exacerbating conditions like multiple sclerosis (MS) (Fresegna, 2020). These side effects are potentially due to the dual role of TNF-α and its receptors, which may have both pro-inflammatory and protective effects depending on the context. Moreover, research exploring the temporal regulation of TNF-α, especially in early versus late stages of disease progression, could provide insights into how the timing of TNF-α modulation may impact treatment outcomes (Guidotti, 2021). As a result, a more thorough understanding of TNF-α’s role in the CNS and further research into the safety and effectiveness of these therapies are necessary to improve treatment strategies.
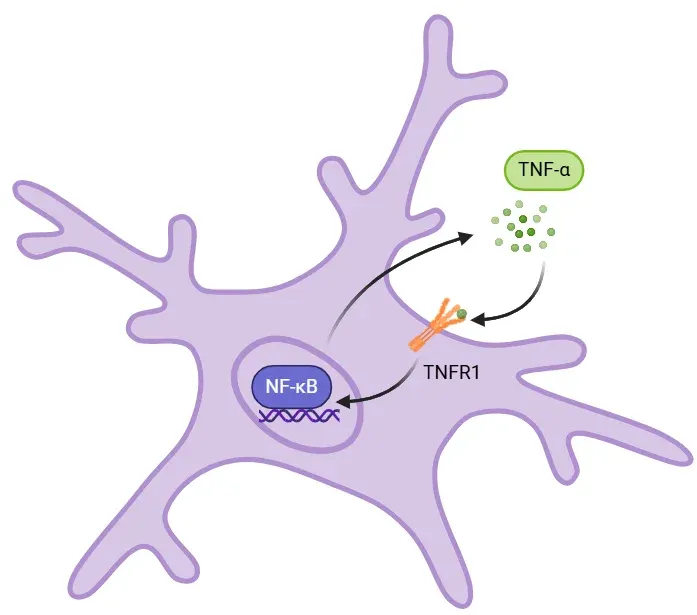
When soluble TNF-α binds to TNFR1, it initiates a signaling cascade (not shown) that activates NF-κB. In the nucleus, NF-κB drives the transcription of proinflammatory cytokines, including IL-6, IL-1β, and TNF-α itself. This cytokine upregulation amplifies the immune response through a positive feedback loop where TNF-α increases NF-κB activity, which, in turn, promotes further TNF-α production. Elevated TNF-α levels can lead to tissue damage, excitotoxity, and other harmful effects. When dysregulated, such as in neurodegenerative diseases, this loop sustains chronic inflammation.
How is TNF-α implicated in neurodegenerative diseases such as AD, PD, and ALS?
Alzheimer's Disease (AD)
AD is a neurodegenerative disease marked by progressive cognitive decline, memory impairment, and the accumulation of amyloid-beta (Aβ) plaques and tau neurofibrillary tangles. Increasing evidence suggests that inflammatory cytokines play a pivotal role in AD pathophysiology. Elevated levels of TNF-α, IL-1β, and IL-6 have been detected in the blood and cerebrospinal fluid (CSF) of patients with AD (Brosseron, 2014). However, some studies have reported conflicting results, potentially due to differences in disease stage. Despite these discrepancies, cytokines like TNF-α tend to increase as AD progresses, although their levels are often too low to serve as reliable biomarkers. The precise relationship between cytokine levels and disease progression remains an area of ongoing research. Some studies suggest that elevated cytokine levels may indicate an increased risk of developing AD, and the ratios of cytokines to their respective receptors may provide more accurate diagnostic markers than individual cytokine levels alone (Brosseron, 2014).
Microglial dysfunction is another critical factor in AD pathogenesis. In models of Aβ accumulation, aged microglia show impaired phagocytic activity and increased expression of inflammatory cytokines, including TNF-α and IL-1β (Hickman, 2008). This dysfunction triggers a feedback loop in which the accumulation of Aβ plaques activates microglia, causing them to release TNF-α. This cytokine release amplifies neuroinflammation and accelerates neuronal damage. Sustained cytokine release promotes further neurodegeneration, which, in turn, worsens the inflammatory response, contributing to increased Aβ deposition. The inability of microglia to effectively clear Aβ plaques results in the accumulation of toxic aggregates. This cycle of plaque buildup, microglial reactivity, TNF-α release, and neuronal damage perpetuates the neurodegenerative process, accelerating the progression of AD (Hickman, 2008).
Clinical trials investigating the therapeutic potential of TNF-α inhibition in AD have yielded mixed results. Small open-label studies showed promising results when the TNF-α inhibitor etanercept was administered via the perispinal route, showing improvements in cognition and behavior (Tobinick, 2008). However, when the same treatment was administered peripherally, no significant changes were observed in these areas (Butchart, 2015). However, a Phase 1b trial for XPro1595, a TNF-α inhibitor that selectively targets soluble TNF-α, has shown promising results in AD (NCT03943264). This new approach, focusing on the soluble form of TNF-α, could offer a more targeted and effective method of modulating the inflammatory response associated with AD.
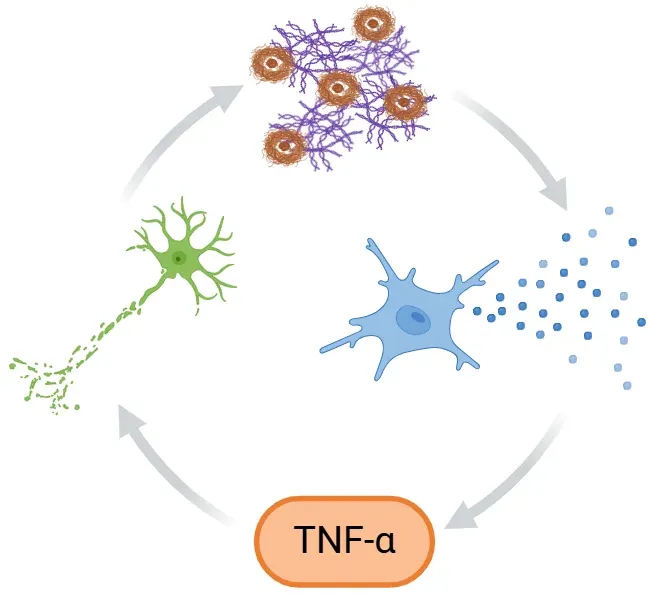
Proposed positive feedback loop in Alzheimer's disease (AD), where the Aβ plaques and tau tangles activate microglia, releasing pro-inflammatory cytokines like TNF-α. This release amplifies neuroinflammation and neurodegeneration, while impaired microglial clearance increases toxic protein buildup, further worsening the cycle and accelerating AD progression.
Parkinson’s Disease (PD)
PD is a progressive neurodegenerative disease characterized by the loss of dopaminergic neurons in the substantia nigra pars compacta (SNpc), leading to motor symptoms such as tremor, rigidity, and bradykinesia. In addition to these hallmark motor symptoms, PD is also associated with a range of non-motor symptoms, including cognitive decline, sleep disturbances, and depression.
Chronic neuroinflammation has been identified as a key factor in the pathophysiology of PD. Postmortem analysis of the SN from PD patients reveals microglial reactivity and elevated levels of pro-inflammatory cytokines, such as TNF-α, which are also found in the serum of PD patients (Collins, 2012). TNFR1 is expressed on dopaminergic neurons in the human SNpc, further suggesting that TNF-α plays a role in the disease process. In addition, animal models of PD show increased TNF-α expression in both serum and CSF (Collins, 2012).
The activation of the transcription factor NF-κB, which regulates genes encoding pro-inflammatory cytokines, is also elevated in PD patients, further linking inflammatory pathways to this disease (Mogi, 2007). Research has shown that chronically reactive microglia release high levels of pro-inflammatory cytokines like TNF-α, which not only damage dopaminergic neurons, but also perpetuate a feedback loop that leads to further microglial reactivity, neuroinflammation, and neurodegeneration in PD (Collins, 2012).
Studies in animal models have demonstrated that blocking TNF-α can significantly attenuate neurodegeneration in PD. For example, treatment with thalidomide, a TNF-α synthesis inhibitor, protects against MPTP-induced dopamine depletion, and TNF-α-deficient mice show a significant reduction in striatal dopamine loss and mortality (Ferger, 2004). Additionally, the use of XENP345, a TNF-α blocker, has been shown to reduce dopaminergic neuron death in murine models of PD (McCoy, 2006). These findings suggest that targeting TNF-α could be a promising therapeutic approach to modulate the inflammatory processes and slow disease progression in PD.
Amyotrophic Lateral Sclerosis (ALS)
ALS is a progressive neurodegenerative disease that primarily targets motor neurons, leading to muscle weakness, difficulty speaking and swallowing, and, ultimately, spasticity and paralysis. In addition to these core features, neuroinflammation plays a significant role in disease progression. Elevated levels of TNF-α have been detected in the plasma and serum of ALS patients, as well as in animal models of the disease (Olmos, 2014; Vu, 2017). Furthermore, the upregulation of NF-κB has been observed in spinal cord microglia from both ALS patients and in the superoxide dismutase 1 (SOD1) transgenic mouse model, a commonly used model for studying ALS (Frakes, 2014). These findings support the involvement of TNF-α in ALS pathophysiology.
To better understand the role of TNF-α in ALS, gene knockout studies have been conducted, particularly in the SOD1 transgenic mouse model. These studies found no significant impact on lifespan or motor neuron degeneration following the genetic deletion of TNF-α (Gowing, 2006). These results suggest that while TNF-α may contribute to the inflammatory processes seen in ALS, it might not be directly responsible for motor neuron degeneration, particularly in the context of SOD1 mutations.
On the other hand, research using TNF-α inhibitors, like thalidomide and lenalidomide, has shown promising results. In SOD1 transgenic mice, these inhibitors improved motor performance and decreased motor neuron cell death (Kiaei, 2006). These results suggest that targeting TNF-α could be a viable therapeutic approach for ALS. However, a Phase 2 clinical trial investigating the use of thalidomide in ALS patients did not replicate these promising results. Daily administration of thalidomide failed to show beneficial effects and was associated with several adverse side effects (Stommel, 2009).
These mixed results may be due to the timing of treatment, as the effectiveness of TNF-α inhibition could vary depending on the stage of ALS progression, highlighting the need for more research to better understand the complex roles of TNF-α in ALS and to refine therapeutic strategies (Guidotti, 2021). While TNF-α inhibition remains a potential avenue for treatment, further studies are essential to determine the most effective methods of targeting this inflammatory pathway in ALS.
In conclusion, TNF-α plays a central role in neuroinflammation and neurodegeneration across diseases like AD, PD, and ALS. Despite differences in their underlying mechanisms, inflammatory processes driven by TNF-α appear to be a common factor in disease progression. While therapies tarting TNF-α show promise in preclinical models, clinical results have been inconsistent, highlighting the need for a deeper understanding of TNF-α’s role. Future research should focus on refining targeted treatments that modulate TNF-α signaling, potentially offering therapies to slow or halt the progression of these neurodegenerative diseases.
What evidence suggests that modulating TNF-α may slow or prevent neurodegenerative diseases?
Given its pivotal role in driving neuroinflammation, TNF-α is central to the progression of many neurodegenerative diseases, including AD, PD, and ALS. Chronic elevation of TNF-α in the CNS leads to neuronal damage and toxic protein accumulation, which together contribute to disease progression. As such, modulating TNF-α has emerged as a promising strategy to slow or even halt the progression of these diseases.
Anti-TNF-α therapies, successful in treating a range of autoimmune and inflammatory diseases, have drawn attention for their potential in neurodegenerative diseases (Gonzalez Caldito, 2023). Drugs such as etanercept (Enbrel®) and infliximab (Remicade®), widely used in treating conditions like rheumatoid arthritis and other peripheral inflammatory diseases, have limited effectiveness in CNS disorders due to poor BBB penetration (Decourt, 2017). Other TNF-α inhibitors, including adalimumab (Humira®), golimumab (Simponi®) and certolizumab pegol (Cimzia®), face similar challenges in accessing the CNS.
This challenge in TNF-α inhibition in CNS diseases is compounded by the risk of serious side effects, such as increased susceptibility to infections and demyelinating diseases (Kemanetzoglou, 2017). For example, in MS, treatments like lenercept have worsened disease outcomes (Maguire, 2021). The dual roles of TNF-α in neuroprotection and inflammation underscore the need for more selective targeting. Research on TNFR1 and TNFR2 has highlighted that selectively targeting TNFR1, while sparing TNFR2, may offer a safer and more effective approach for treating MS (Fresegna, 2020).
In response to these limitations, development of selective TNF-α inhibitors that specifically target TNFR1 for CNS use, such as atrosab and atrosimab, have demonstrated promising results in preclinical models of neuroinflammation, such as experimental autoimmune encephalomyelitis (EAE) (Richter, 2021). Similarly, a Phase 1b trial for XPro1595, a TNF-α inhibitor selective for soluble TNF-α has shown promising results in AD (NCT03943264). These therapies aim to offer a more targeted approach, improving safety and efficacy for neurodegenerative diseases.
While preclinical findings are promising, further clinical trials are necessary to establish the safety and effectiveness of these selective TNF-α blockers in humans. These studies will be crucial for determining whether these therapies can provide benefits for patients with neurodegenerative diseases. As research continues, the development of TNF-α therapies tailored for the CNS may offer an important approach to slowing or preventing neurodegeneration, improving outcomes for patients and advancing the field of neuroinflammation treatment (Decourt, 2017; Hampel, 2020; Zahedipour, 2022).
Our team would be happy to answer any questions about the function of TNF-α in microglia and its contribution to neurodegenerative diseases or provide specific information about the AD, ALS, and PD models we use for therapeutic efficacy studies.
Discover more about our Neurodegenerative Diseases Models
Related Content
Up-to-date information on TNF-α and microglia in neurodegenerative diseases and best practices related to the evaluation of therapeutic agents in animal models of neurodegenerative diseases.
Microglia, Astrocytes & Tau in Neurodegenerative Diseases
How glial-driven neuroinflammation fuels tau aggregation, propagation, and neuronal loss in Alzheimer’s disease and other tauopathies.
Microglia, Astrocytes & α-Synuclein in Parkinson’s Disease
How α-synuclein influences microglia and astrocytes in Parkinson’s disease and other synucleinopathies.
Mitochondrial Dysfunction in Microglia & Astrocytes
The role of mitochondrial dysfunction in microglia and astrocytes in neurodegenerative diseases, including Alzheimer’s disease, Parkinson’s disease, and ALS.
Microglial Senescence and Neurodegenerative Diseases
An overview of microglial senescence and its role in neurodegenerative diseases, including Alzheimer’s disease (AD) and Parkinson’s disease (PD).
Microglia Morphology in ALS, Alzheimer's Disease & Parkinson's Disease
An overview of microglial morphological analysis and the applications to neurodegenerative disease research and drug discovery & development.
Amyloid-β & Inflammatory Microenvironment in Alzheimer's Mice
We have analyzed the complex spatial relationships between β-amyloid plaques, activated & resting microglia, and astrocytes in an APP/PS1 transgenic model.