What is the EAE MS model?
Experimental Autoimmune Encephalomyelitis (EAE) is the most widely used animal model of multiple sclerosis (MS). EAE replicates several key aspects of human MS, including peripheral inflammation & neuroinflammation, demyelination, and axonal injury. The infographic below highlights several of the key features of EAE in mouse and rat models.
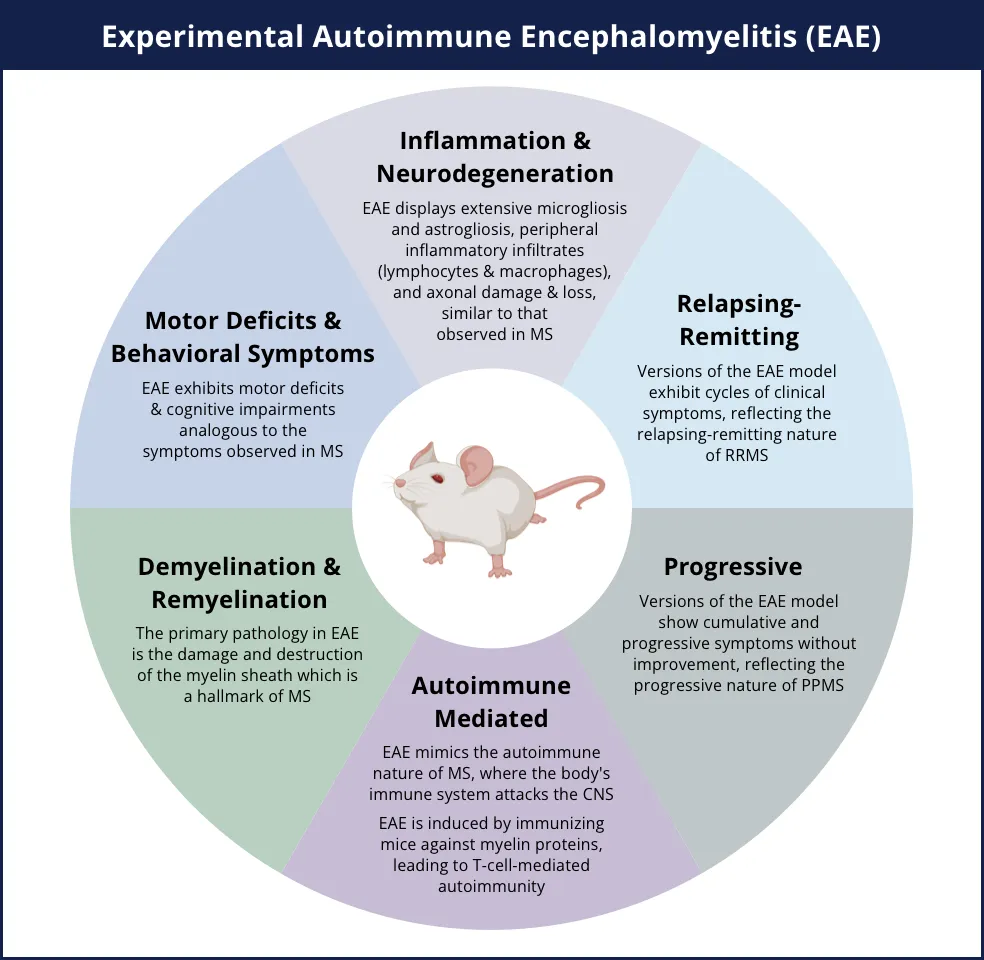
EAE models key aspects of human multiple sclerosis (MS), including autoimmune-mediated disease, demyelination & remyelination, inflammation & neurodegeneration (including axonal injury), motor deficits, and relapsing-remitting and/or progressive disease courses.
Click to copy link
EAE can be induced in mice or rats either by active immunization with myelin proteins or peptides (e.g. MBP, MOG, PLP) in an adjuvant, or by the passive transfer of activated myelin-targeted CD4+ T cells. The primary manifestations of EAE occur in the spinal cord, characterized by infiltration of T lymphocytes and monocytes, leading to localized inflammation and the development of demyelinating lesions.
EAE effectively demonstrates the translational potential of rodent models, as several MS drugs currently on the market were initially tested using this model. For instance, glatiramer acetate (GA; Copaxone) and natalizumab (Tysabri), a monoclonal antibody against VLA-4 integrin, and fingolimod (FTY-720, Gilenya), a sphingosine-1-phosphate (S1P) receptor modulator, were evaluated in EAE models.
How is axon damage typically measured on tissue sections from EAE models?
Axon degeneration and injury can be measured on tissue sections from the spinal cords of EAE mice and rats. By performing quantitative image analysis of brightfield immunohistochemistry staining or multiplex immunofluorescence staining, quantitative data from different regions of interest (e.g. gray matter, white matter) at multiple levels of the spinal cord (e.g. cervical, thoracic, lumbar) can be obtained. Common IHC/IF markers of axon integrity/injury include phosphorylated and non-phosphorylated neurofilaments, as well as amyloid precursor protein (APP). Some illustrative examples of the use of these markers in EAE models are provided below.
Overview of the process for measuring axonal damage on tissue sections from EAE rodent models.
Aharoni and colleagues stained spinal cord tissue from MOG35-55 EAE mice for neurofilament light, medium, and heavy proteins. They found that extensive axonal deformation and loss are evident in sites of demyelination. When these mice were treated with glatiramer acetate, the axonal damage/loss and demyelination was reduced. Budde et al. used quantitative analysis of SMI-32 staining for non-phosphorylated neurofilaments as a marker of axon damage. They found an association with axial diffusivity measured by in vivo diffusion tensor imaging (DTI) MRI in the spinal cord of EAE mice.
Herrero-Herranz and colleagues investigated immunoreactivity for APP, which has been described as a marker for acute axon injury, in MOG-EAE mice. They found that many axons were APP-positive early in the disease course, but significantly decreased in later disease stages. In this study, the investigators also stained spinal cord tissue sections with SMI-31 for phosphorylated and SMI-32 for non-phosphorylated neurofilaments. In the early phase of MOG-EAE, SMI-32 positive axons increased, while the percentage of SMI-31 positive axons decreased in the lesion. At the later stages of disease progression, a large increase in SMI-32 positive axons with only few SMI-31 positive axons was observed, thereby indicating that the relative amount of phosphorylated neurofilaments decreases, while non-phosphorylated neurofilaments increase over the course of MOG-EAE.
Can neurofilament light chain (NfL; NF-L) levels in the blood & CSF be used to assess axonal injury in EAE?
Several groups have demonstrated the use of plasma and CSF neurofilament light chain (NfL) levels as fluid biomarkers of axonal injury in EAE. Measurement of biological fluid concentrations of NfL is attractive in EAE mice given that it shows promise in human MS studies, including MS clinical trials, thereby serving as a potential translational biomarker.
At Biospective, we routinely measure NfL levels in the plasma and CSF in MOG35-55 EAE mice as part of our therapeutic efficacy studies. As shown in the figure below, NfL is significantly elevated starting around peak disease (as measured by the EAE clinical score).
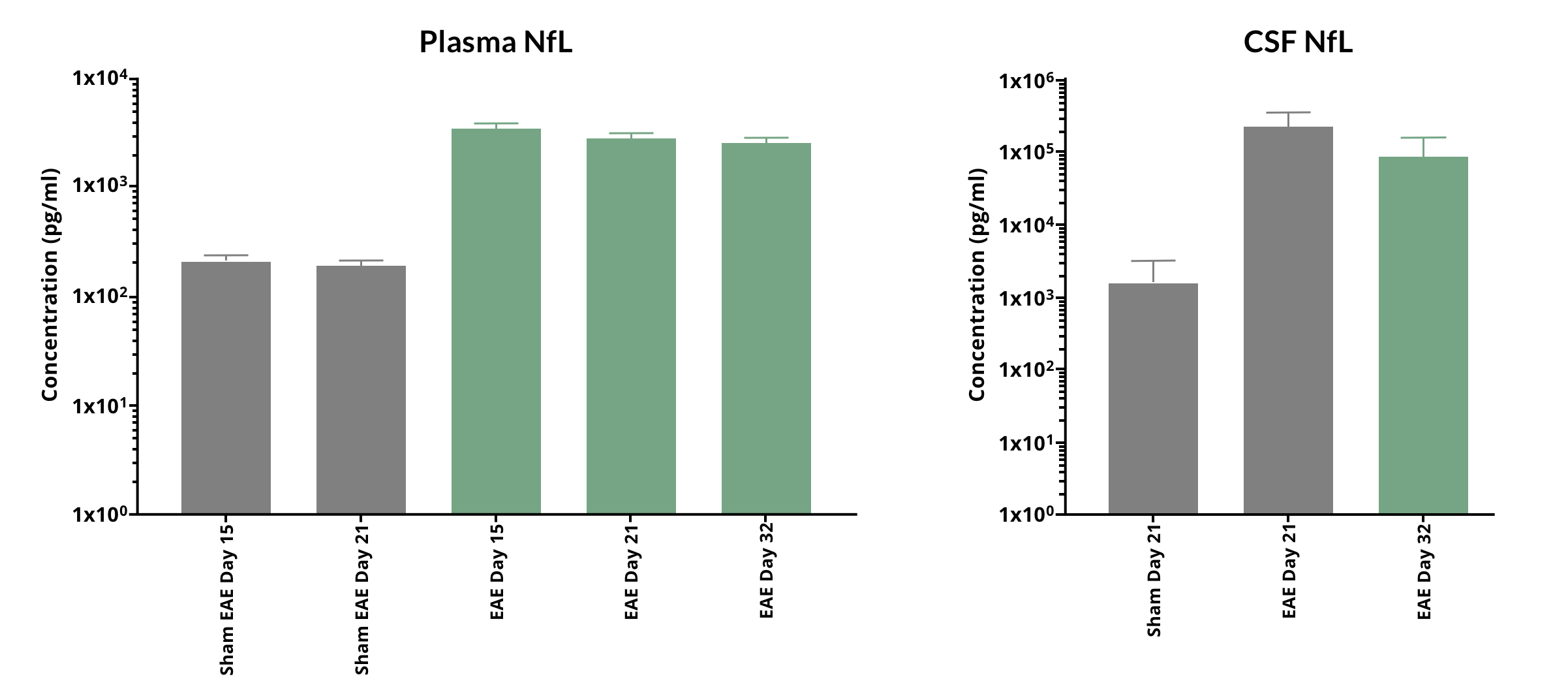
Highly elevated levels of NfL (mean ± s.d.) are found in the plasma and CSF of MOG35-55 EAE mice over the disease course.
Kieseier et al. performed a meta-analysis of twenty-five independent mouse EAE studies conducted over two years. In C57BL/6J mice with EAE induced via rat MOG28-152 in complete Freund’s adjuvant, they showed that plasma and CSF NfL levels significantly increased following disease induction. These levels remained elevated up to one month and showed an approximately 10-fold reduction at two months post-disease induction. They also found that correlations between plasma NfL and CSF NfL levels, as well as between the plasma or CSF NfL levels and EAE clinical scores, were highly significant.
Aharoni et al. investigated neurodegenerative changes in the MOG-induced EAE mouse model and the effect of glatiramer acetate (GA) [Copaxone] treatment on these manifestations. The found early onset of axonal damage in this model, as revealed by a detailed kinetic analysis of neurofilament light chain leakage into the blood as a function of disease duration, as well as by immunohistochemistry (IHC) analysis.
Brummer and colleagues found that a spatiotemporal patten of release of NfL into the blood in MOG-EAE mice can be driven by CNS-myeloid cells (CMC), such as microglia and infiltrating macrophages, and may progress in a disease stage-dependent centripetal manner from normal appearing white matter (NAWM) to white matter lesions (WML). They also demonstrated that serum neurofilament light chain (sNfL) levels were associated with the respective anatomical lesion location, which may provide a pathophysiological explanation for the high inter-individual variance of sNfL levels in MS patients.
Norgren et al. induced EAE in dark Agouti rats using recombinant MOG1-125, and found a highly significant statistical difference in the CSF NfL levels between the control group and the diseased rats. Similarly, Zuo and colleagues found that passive EAE, induced by an adoptive transfer of young Th17 cells in SJL/J mice, generated a non-remitting clinical phenotype that was associated with increased levels of serum NfL at peak disease in young and old mice. Further, at the post-acute phase of disease, the old mouse recipients exhibited increased NfL compared with sex-matched young mouse recipients, and the serum NfL levels were positively correlated with the disease severity measured by the EAE score.
Zahoor et al. investigated the profile of blood-based analytes including, cytokines (IL6, IL17, IL12p70, IL10, and TNFα) and neural markers (NfL and GFAP) in the plasma of relapsing-remitting (RR) (SJL mice induced with the PLP139–151 peptide) and chronic (B6 mice induced with MOG35–55) mouse models of EAE during different phases (acute, chronic, and progressive) of disease course using the Simoa assay. Plasma NfL (as well as GFAP) showed a substantial increase during post-disease onset at peak, chronic, and progressive phases in both the RR SJL and chronic B6 mouse EAE models.
Our team would be happy to answer any questions about EAE models or provide specific information about the models that we use for therapeutic efficacy studies.
Discover more about our MS Models
Related Content
Up-to-date information on Multiple Sclerosis and best practices related to the evaluation of therapeutic agents in MS animal models.
Demyelination & Remyelination in the Cuprizone Model
An overview of the methods available to measure myelin and oligodendrocytes in the cuprizone demyelination mouse model of multiple sclerosis (MS).