This resource describes:
What is the cuprizone-induced demyelination model of multiple sclerosis?
Multiple sclerosis (MS) is a prevalent, chronic, autoimmune demyelinating disorder of the central nervous system (CNS), affecting over 2.8 million people globally (Walton, 2020). In MS research, various preclinical models are used to replicate different aspects of the disease, including demyelination models, such as lysolecithin (LPC) mouse & rat models and the cuprizone mouse model (Dedoni, 2023).
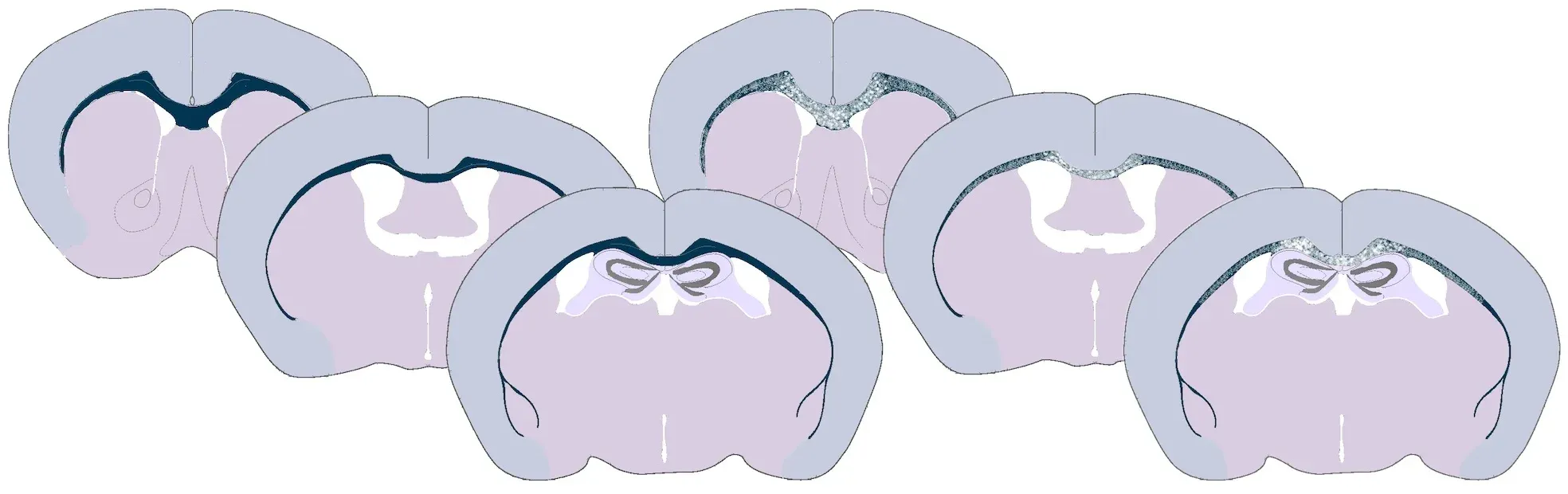
Illustration of demyelination in the corpus callosum of cuprizone-fed mice. A normally myelinated corpus callosum is shown on the left and the partially demyelinated white matter tract is shown on the right.
The cuprizone model is a widely-used toxin-induced multiple sclerosis model (Kipp, 2024; Zhan, 2020). Cuprizone (C14H22N4O2), or oxalic acid bis [cyclohexylidene hydrazide], is a copper-chelating agent that, when administered orally, induces the death of mature oligodendrocytes and subsequent demyelination of specific white matter tracts in the rodent brain (Dedoni, 2023). This model involves the activation of innate immune cells within the brain, but T and B lymphocytes do not play a central role (Wolf, 2018), thereby distinguishing it from other MS models that involve the adaptive immune system, such as Experimental Autoimmune Encephalomyelitis (EAE), Theiler’s Murine Encephalopathy Virus (TMEV), and other viral models of MS (Dedoni, 2023). Cuprizone-induced demyelination is not lymphocyte-dependent and occurs even in lymphocyte-deficient recombination activating gene (Rag) knockout mice (Hiremath, 2008).
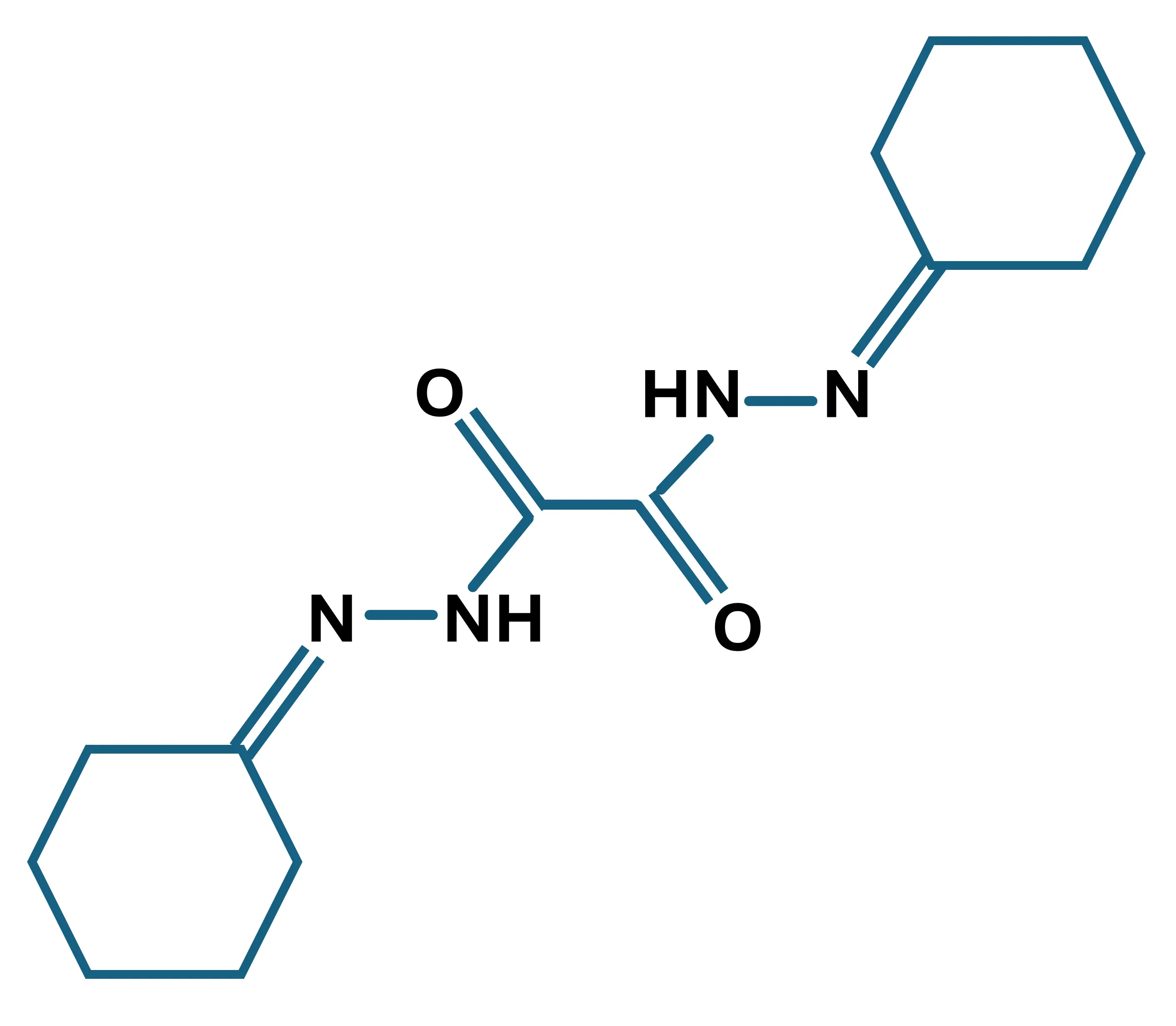
Molecular structure of cuprizone; C14H22N4O2; molecular weight: 278.45 g/mol
The cuprizone model allow researchers to dissect and investigate specific mechanisms in the progression of MS. This rodent model can be divided into two phases: (1) initial demyelination in the corpus callosum and other brain regions, such as the superior cerebellar peduncles and cerebral cortex (Vega-Riquer, 2019), which is accompanied by activation of the innate immune system, and (2) significant remyelination of exposed axons once cuprizone treatment is terminated (Gharagozloo, 2022).
In practice, mice are fed cuprizone for 5-6 weeks. This approach leads to selective oligodendrocyte stress and death, resulting in acute demyelination of distinct brain subregions, extensive microglial activation and astrocytic proliferation, a breakdown of the axonal transport mechanisms (Rühling, 2019), and, in more chronic models, overt axonal damage (Lindner, 2009). The exact mechanisms behind oligodendrocyte degeneration are not fully understood, but may include mitochondrial disruption due to a cuprizone-induced copper deficit, ferroptosis (Jhelum, 2020), and endoplasmic reticulum stress responses.
The timeline of cuprizone's effects is well-documented. In the early phase, within the first week of treatment, oligodendrocyte death can be observed as early as two days after treatment begins. There is early activation of astrocytes (Kipp, 2023) and microglia (Gudi, 2014), followed by rapid loss of mature oligodendrocytes, with approximately 65% lost by the second day and 80% by the end of the first week (Kipp, 2023). In the intermediate phase, spanning 1 to 3 weeks, the loss of oligodendrocytes and activation of glial cells continue, with signs of demyelination and acute axonal pathology becoming evident (Crawford, 2009; Kipp, 2023).
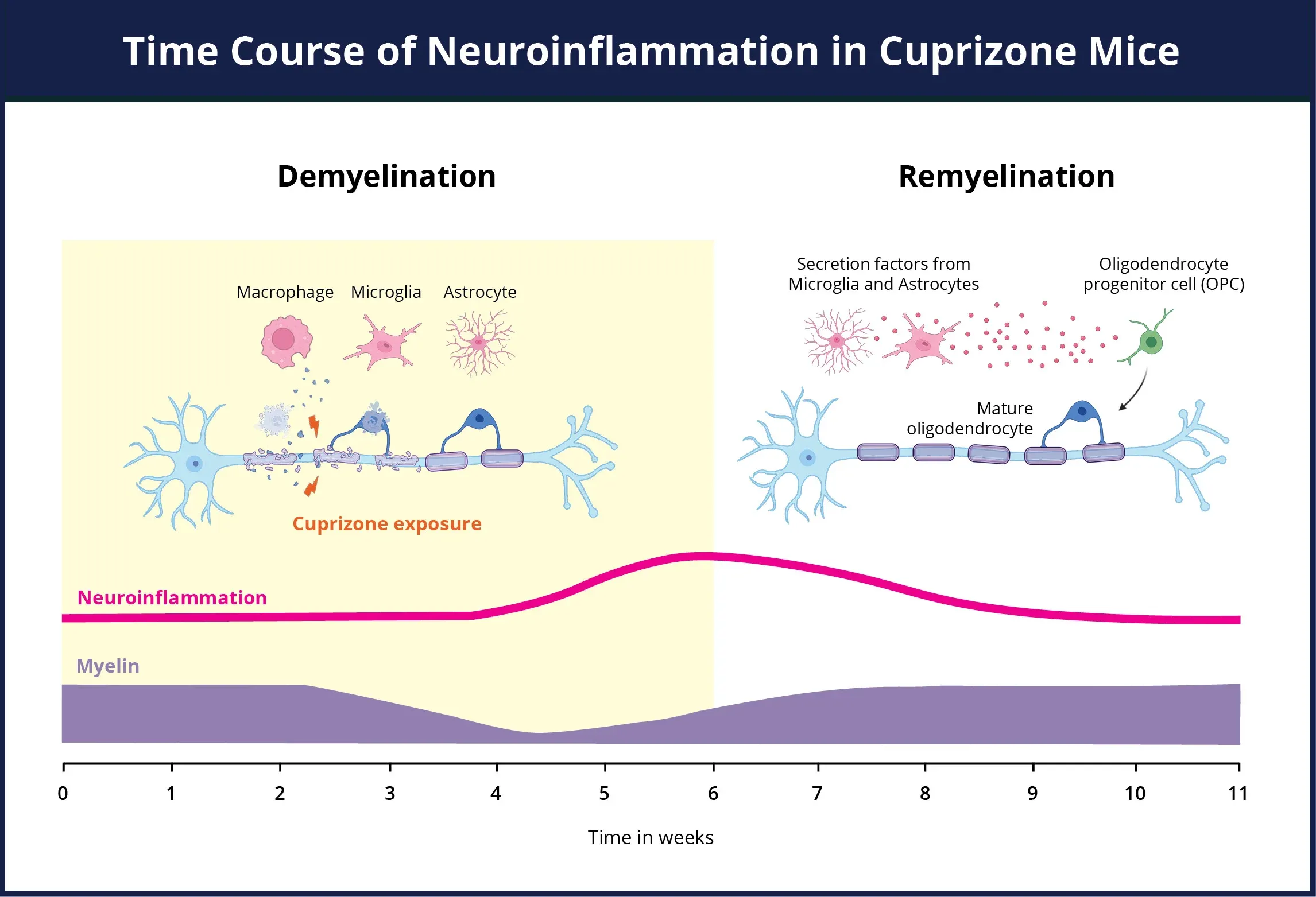
An overview of the neuroinflammation time course for the cuprizone model. During the cuprizone exposure period, there is a concurrent loss of myelin (purple) and a dramatic elevation in the populations of activated microglia & reactive astrocytes (pink) contributing to neuroinflammatory state. Both astrocytes and microglia play an active role in the removal of myelin debris, as well as the activation of the oligodendrocyte progenitors. Upon removal of the cuprizone insult and the remyelination of the axons, the neuroinflammation resolves.
Click to copy link
Between 3 and 5 weeks, demyelination becomes visible, and OPCs are activated and recruited to replace the lost oligodendrocytes (Brousse, 2015). Impaired motor coordination and behavioral deficits, primarily in spatial memory and social behavior, become evident (Franco-Pons, 2007). At this stage, if the cuprizone-containing diet is replaced with a standard diet, the demyelination process stops and remyelination occurs.
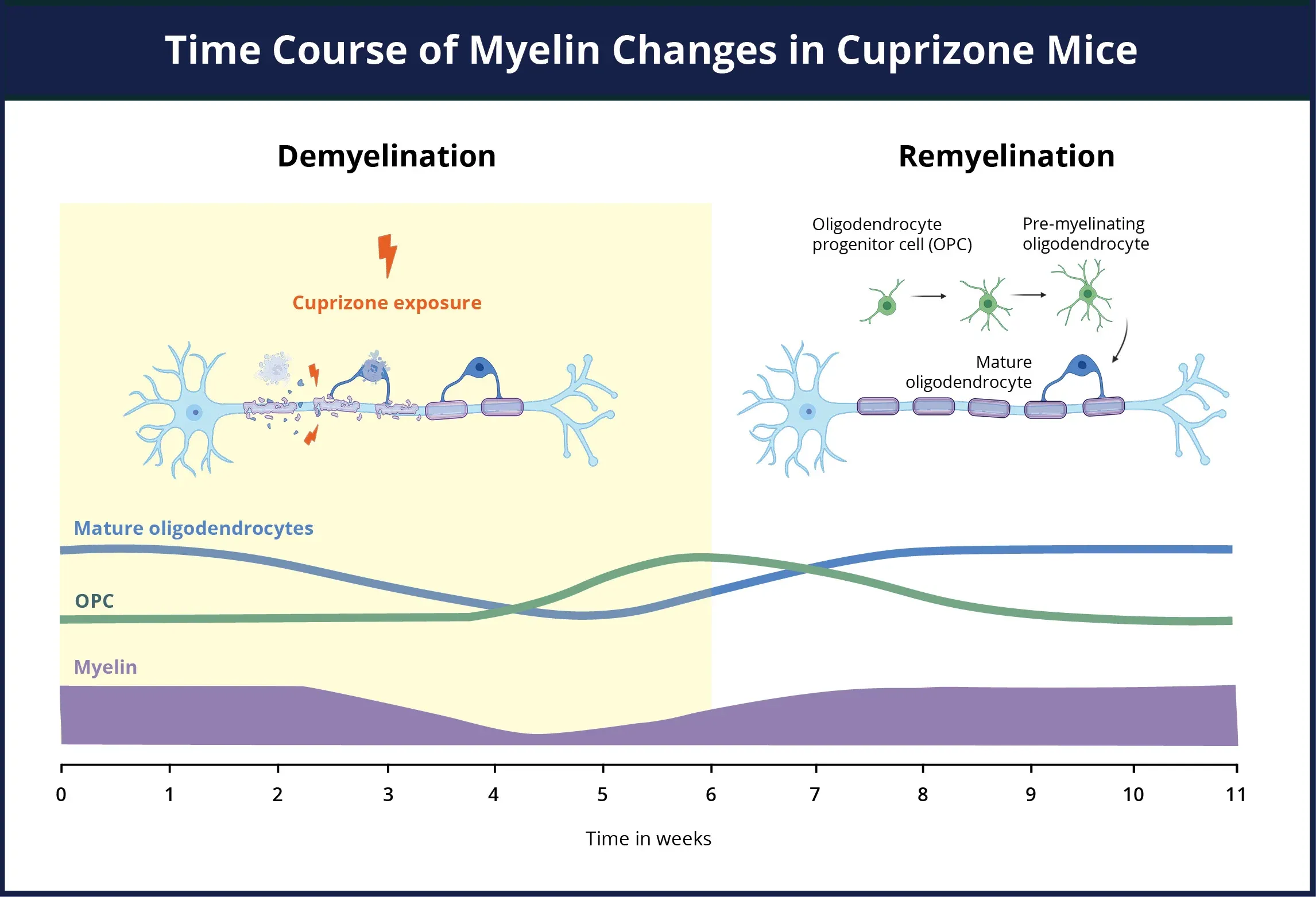
An overview of the demyelination and remyelination timeline for the cuprizone model. During the cuprizone exposure period, there is a concurrent loss of myelin (purple), death of mature oligodendrocytes (blue), and a dramatic elevation in proliferation and differentiation of oligodendrocyte progenitors (green) into pre-myelinating oligodendrocyte precursor cells (OPCs). The OPCs migrate and differentiate at the site of the tissue damage even while there is still cuprizone present. Upon removal of the cuprizone insult, the OPCs rapidly differentiate into mature oligodendrocytes, which begin remyelinating the exposed axons.
Click to copy link
If the treatment is prolonged for over 12 weeks, the demyelination can become chronic, with the OPC population becoming exhausted and the potential for remyelination profoundly diminished (Lindner, 2009). Numerous studies have illustrated that the extended exposure to cuprizone induces chronic lesions that show a limited endogenous remyelination capacity (Leo, 2022), prolonged astrogliosis (Hibbits, 2012), and more extensive axonal damage (Lindner, 2009). In summary, the cuprizone model is a crucial tool in MS research as it replicates key aspects of the disease, particularly oligodendrocyte degeneration and resulting demyelination. Understanding the timeline and mechanisms of cuprizone-induced damage is essential for developing targeted therapeutic interventions for MS.
What are the most common methods for assessing demyelination & remyelination in cuprizone mice?
To effectively study and understand the processes of demyelination and remyelination, it is crucial to employ reliable techniques for detecting myelin and the demyelination & remyelination processes within the central nervous system (CNS). Visualization of myelin in experimental tissues can be accomplished with histological & immunohistochemistry (IHC) techniques. Ultrastructural analysis of myelin sheaths can be visualized by electron microscopy (EM), providing high-resolution images. Advanced, non-invasive in vivo imaging techniques, such as magnetic resonance imaging (MRI), can provide insight into the ongoing process. While each of these techniques can facilitate a deeper understanding of myelin-related disorders, they each have pros and cons as outlined below.
Histological Staining
There are a variety of histochemical techniques available for evaluation of demyelination and remyelination in cuprizone mice. A few commonly used staining methods are highlighted below.
Luxol Fast Blue (LFB)
Luxol Fast Blue (LFB) is a rapid histological stain used to provide insights into myelination in tissue sections. It effectively reveals clear voids where axonal tracts have been demyelinated, and is useful to identify the extent of myelin damage. The LFB method involves over-staining the tissue, followed by differentiation in lithium carbonate to distinguish between myelinated and demyelinated areas. The differentiation steps must be tailored to the specific tissues to achieve optimal staining. However, this process can generate significant batchwise variability, making LFB a poor candidate for quantitative analysis.
FluoroMyelin™ and other Myelin-binding Fluorescent Stains
FluoroMyelin™ stains are commercially available fluorescent dyes that have selectivity affinity for myelin. These dyes have been developed in a number of different fluorescent colors that can be used to visualize myelin in combination with other markers within brain tissue sections. Numerous other fluorescent compounds that have an affinity for myelin have also been developed. The disadvantages of these fluorescent myelin binding compounds include a tendency to generate strong background staining, and showing low-staining contrast and often low brightness, thereby making them challenging to use in quantitative multiplex staining.
Silver Staining
Silver staining is a very sensitive technique used for visualizing tissue structures, but it is a laborious procedure that requires tailored development times, leading to significant batchwise variability. Although it is highly sensitive, silver staining has a very low dynamic range and tends to stain various other proteinaceous structures, making it a poor candidate for quantitative analysis.
Toluidine Blue
Toluidine Blue O is an anionic histological dye that binds to myelin (among other structures) and allows for detailed visualization of myelin wrapping. It is often used for peripheral nerves, and, while challenging, can be used within the CNS. The tissue preparation is laborious, as the process requires EPON resin embedding, and is most effective when staining semi-thin (0.5-1 μm) plastic-embedded tissue sections. Visualization of myelin within the corpus callosum requires detailed dissections, as the process is optimal in small tissue samples and sections must be perpendicular to the axons to visualize the myelin sheaths effectively. A disadvantage of this method is that it is time-consuming and does not allow for immunostaining of the same tissue. Additionally, Toluidine Blue O stains will bind to other structures, making careful tissue sampling crucial.
Immunohistochemistry (IHC) Staining
Immunohistochemical staining for myelin using markers, such as Myelin Basic Protein (MBP), Proteolipid Protein (PLP), and Myelin Oligodendrocyte Glycoprotein (MOG), on tissue sections is highly effective for identifying the myelin sheath associated with mature oligodendrocyte processes and cell bodies. This technique is excellent for quantifying myelin levels and visualizing the extent of myelin damage, but is not ideal for enumerating the number of myelin-producing cells.
Immunohistochemical staining for oligodendrocyte lineage markers, such as Olig2, PDGF-α-Receptor (PDGFRα), and NKX2.2, provide significant insights into the maturation and differentiation stages of oligodendrocyte precursor cells (OPCs). These markers are often localized in the nucleus or cell body, making them ideal for counting and tracking cell numbers during the OPC maturation and differentiation process. Olig2 is a transcription factor critical for involved in the proliferation and differentiation of oligodendrocytes. It is expressed in the nucleus of OPCs and continues to be expressed in mature oligodendrocytes. PDGFRα is a cell-surface receptor that binds platelet-derived growth factor (PDGF). It is a marker of early OPCs and is important for their proliferation and survival. PDGFRα expression is typically downregulated as OPCs differentiate into mature oligodendrocytes. NKX2.2 is a transcription factor that plays a crucial role in the differentiation of OPCs into mature oligodendrocytes. It is expressed in the nucleus and is involved in the regulation of genes necessary for oligodendrocyte maturation and myelination.
Immunohistochemical staining for Myelin Basic Protein (MBP) in multiple tissue sections, highlighting demyelination in the midline of the corpus callosum in a mouse exposed to cuprizone diet for a period of five weeks.
Electron Microscopy (EM)
Electron microscopy offers ultra-high resolution images of tissue samples, providing exquisite details of myelin structure and wrapping at both the cellular and subcellular levels (Cruz-Martinez, 2016). It is utilized to determine the g-ratio of myelinated axons, and is an excellent technique for distinguishing developmentally myelinated axons from remyelinated axons. However, it samples only very small regions of the tissue, and in the cuprizone model, does not facilitate the insight into the extent of demyelination or remyelination throughout the corpus callosum.
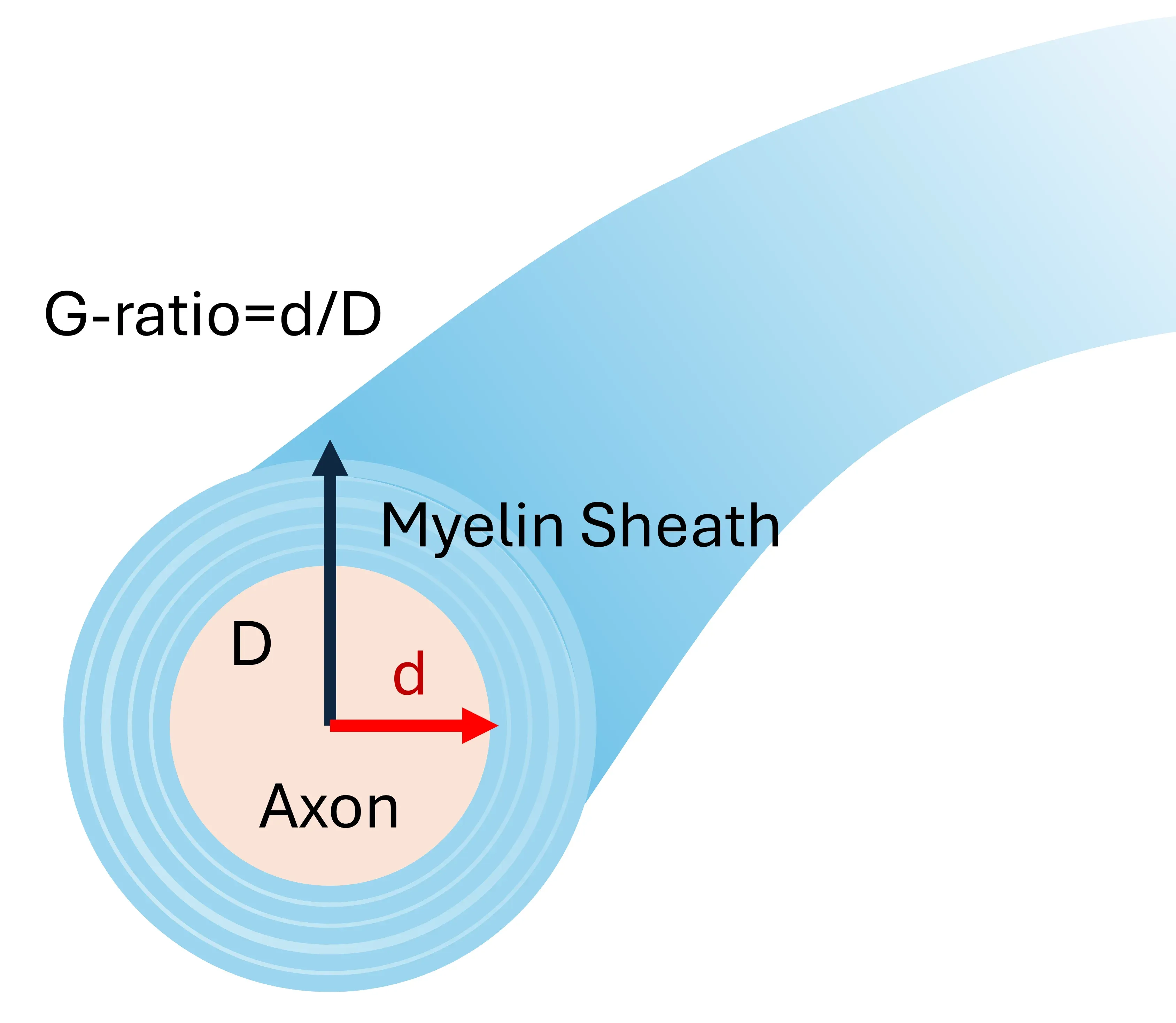
lllustration of the g-ratio calculation
In Vivo Imaging
Preclinical Magnetic Resonance Imaging (MRI) and Diffusion Tensor Imaging (DTI) allow for structural assessment due to the tissue-specific nature of water diffusion. Myelinated axons exhibit a characteristic constrained and directional DTI signal (Wang, 2019). These techniques can be performed as in-life assessments, providing insights into the evolution of myelin changes and are fully translatable (i.e. translational biomarkers) from preclinical efficacy studies to clinical trials (Sommer, 2022).
Magnetization Transfer Ratio (MTR) (Tagge, 2016) is another MRI technique that provides insights into myelin content by measuring the transfer of magnetization between free water and macromolecule-bound water. This method, like DTI, can be performed as an in-life assessment, offering valuable information on the evolution of myelin changes. MTR is also translatable from preclinical efficacy studies to clinical trials (Sommer, 2022).
While powerful tools for non-invasive, longitudinal, in vivo assessment, MRI, DTI, and MTR do not allow for microscopic assessment and can be confounded by edema, although this issue is not a particular consideration for the cuprizone model given that vasogenic edema does not play a prominent role.
What are practical considerations for measuring demyelination & remyelination in preclinical therapeutic efficacy studies?
Important considerations when utilizing the cuprizone model for preclinical therapeutic efficacy studies as part of drug development studies include determining the appropriate time window for both the intervention and the endpoint readouts. The timing of intervention needs to take the pharmacokinetics (PK) of the therapeutic agent and the targeted cell type into consideration.
Often a therapeutic effect will change the rate of the demyelination and remyelination phase, while ultimately the model will resolve to a very similar final remyelinated state. As such, selecting the most appropriate timing for endpoint assessments is also crucial. Given that demyelination and remyelination processes occur over different time frames, multiple time points should be evaluated to capture the dynamics of these processes.
Choosing an appropriate pathological assessment is essential to generate objective and reproducible quantification. Ensuring that tissue preparation and sectioning allow for targeted localization of the pathology to the regions of the corpus callosum most impacted is important for cross-group comparisons.
Maximizing insight into the evolution and resolution of the demyelinating & remyelinating lesion can be achieved by utilizing in vivo imaging. Techniques such as diffusion tensor imaging (DTI) and magnetization transfer imaging (MTR) provide insights into white matter integrity. Longitudinal studies using MRI allow for repeated measures in the same animal, thereby enabling the tracking of disease progression and therapeutic effects.
Our team would be happy to answer any questions about the Cuprizone Mouse Model or provide specific information about the MS models that we use for therapeutic efficacy studies.
Discover more about our MS Models
Related Content
Up-to-date information on Multiple Sclerosis and best practices related to the evaluation of therapeutic agents in MS animal models.
Experimental Autoimmune Encephalomyelitis (EAE) & Axonal Injury
This resource describes the methods available for measuring axonal damage & axon degeneration, including tissue markers and plasma & CSF neurofilament light chain (NfL; NF-L) levels, in the EAE model of multiple sclerosis (MS).